BACKGROUND
Athletic Performance
The most commonly considered factors, which determine physical performance, are of peripheral nature: muscle strength and cardio-respiratory capacity. These are influenced largely by genetic factors and by specific training. However, it is unlikely that these factors alone decide the outcome of a sporting competition with often only milliseconds or one point between a first and a second place. The determinants of athletic performance are complex and thus must involve top down control mechanisms regulating motor performance.
For decades the brain was not considered to play an important role in determining athletic performance. The original model of factors limiting human exercise performance was proposed by Hill in 1924 suggesting that performance declines when oxygen requirements of the exercising muscles exceed cardiac output capabilities and therefore have to function anaerobically, producing excess lactic acid, which in turn impairs muscle contractions.1 This model states, that a disruption of homoeostasis is the cause of exercise termination. Although a decrease in voluntary muscular contractility plays a role in sports performance, this point is rarely reached during intense training and even competition, especially in the endurance domain.
Theories like Hill’s “Catastrophe model” dominated the field of exercise and performance science for over half a century. Many exercise physiologists have noted early on, that exercise capacity is most likely limited by an integration of multiple factors. To reach the highest level of performance all systems have to be optimized, from muscles, lung and heart, all the way to the highest control-unit: the brain. If the brain as the central control system does not function appropriately, the remaining systems cannot perform at their best.
Today most exercise physiologists, athletes and coaches agree that performance decreases with increasing fatigue. The term fatigue is commonly split into two components: peripheral and central fatigue. While peripheral fatigue is broadly defined as a reduction of muscular force which is easily measurable, central fatigue is often described as a sensation or an emotion which is more difficult to quantify.2 There is currently no consensus on a clear-cut definition of fatigue, however attempts of clarification have been made,3 including – in the context of sports performance – the inability to meet a force expectation, which is the inability to generate maximal force or a slowdown in building up of force.4
Models of Fatigue
Different theories and models to explain fatigue in endurance sports currently exist and include the Central Governor Model (CGM),5 critical body temperature6 and the neurohumoral response also called Central Fatigue Hypothesis (CFH).7 The CGM suggests the brain as a regulator of muscle performance, thus including factors like motivation, presence of competitors, knowledge of end point, deception and self-belief.8 The anticipatory regulation of exercise performance is what distinguishes it from the CFH, which suggests that fatigue occurs as a result of changes in neurotransmitter concentration in the brain.9 The most prevalent theory about the neurohumoral basis of Central Fatigue (CF) is the Trp-5-HT-hypothesis developed by Newsholme and others,10 which is based on disturbances in brain serotonin (5-HT) levels.
Fatigue can be caused by processes anywhere along the brain-muscle pathway and viewing peripheral and central mechanisms as separate entities will not further our understanding of fatigue as a whole. Viewing the CGM and the CFH not as mutually exclusive, but rather as complementary may be the key to unravel the mechanism determining exercise performance.
Placebo Effect and Ergogenic Aids
An indicator for the important role of the brain in limiting or enhancing exercise performance is that it can be influenced by expectancy effects caused by beliefs about the efficacy of ergogenic aids.11,12,13 An ergogenic aid is any form of mechanical, pharmacological, physiological, psychological or nutritional aid that enhances exercise performance. In the past decade several studies suggested a measurable placebo effect in exercise performance, which is not substance specific and is significantly influenced by factors such as personality of the athlete and perceptual characteristics of the ergogenic aid.14 This has first been shown in the early 1970’s in weight lifters, whose performance increased significantly when they were falsely told that they took anabolic steroids.15 Results of such expectations and believes are commonly known as placebo effects and are defined as the improvement of symptoms due to the administration of an inert treatment.
The underlying neurobiological or psychophysiological change caused by a placebo manipulation is called placebo response. The placebo response can contribute to the efficacy of any active treatment16 and can be triggered by the patients’ expectations, the relationship to the physician/trainer or by behavioural conditioning.17,18,19,20 The placebo effect is an empowering concept, because it provides a scientific basis for the influence of the psychosocial context on treatment outcomes and reveals how internal control processes shape perception, emotions and motivation and vice versa.21
Several studies have demonstrated that athletes who believed that they had ingested carbohydrates (CHO),22 caffeine23,24 or had used a respiratory training device25 showed performance enhancement. A review of 12 studies looking at sports performance changes following interventions noted placebo responses between -7.8% and +50.7%.26 In 2008, Pollo, et al. were able to show that placebo-induced expectations of better performance can reduce the perception of fatigue while increasing muscle work, suggesting top-down modulation of exercise performance via central fatigue mechanisms.27 A recent study investigated elite athletes’ attitudes toward placebo induced performance enhancement and concluded that roughly 50% of elite athletes have experienced placebo effects, the majority believes in the possible ergogenic effect of placebos and those with past placebo experiences would not mind them being more widely used.28
Studies of the placebo response of nutritional supplements have looked at specific isolated substances such as glucose,29,30 amino acids,31 caffeine,32 alcohol33 and ginseng.34 By general definition, nutritional supplements are “[…] products that are taken orally with the intention to supplement the diet by increasing the total dietary intake of vitamins and minerals and non-vitamin non-mineral substances”.35 The placebo response evoked through the consumption of a nutritional supplement administered in the form of food, which stimulates a multitude of senses may, however, be proportionally larger due to increased stimulus salience. The association of physiological changes and specific flavours was shown in studies eliciting immune responses via classical flavour conditioning36,37 and in studies influencing the severity of motion sickness via Pavlovian flavour conditioning.38 A qualitative study with 267 undergraduate college students compared the perceived effectiveness in enhancing strength, endurance and concentration of nine fictive nutritional supplements differing in shape (e.g. drink, lotion, pill, capsule, bar, powder), colour (e.g. green, white, red) and mode of administration (drink, swallow, application to skin). The results showed that the perceptual characteristics of nutritional supplements influence the expectation of their consumers regarding their potency, however there seems to be large inter-individual variability.39 The mode of administration of a CHO supplement was analyzed for its influence on the metabolic response and exercise performance and researchers found no difference in the beneficial effects of sport beans, sport drink or gel.40 These results however could be due to a ceiling effect, meaning that the administered dose of CHO reached the level above which variance in endurance performance is no longer measurable, thus masking any potential influence of perceptual attributes. Including placebo groups receiving the same supplements matched for flavour and appearance without CHO could have revealed any potential differences.
Looking at nutritional supplements as ergogenic aids, in the context of exercise performance, provides a way to quantify the associated placebo response via both objective and subjective performance measures and thus provides access to the investigation of central control mechanisms of motor performance.
Neural Basis of Motor Performance
The neural basis of placebo induced performance changes have only recently been investigated in detail. Most knowledge so far has come from studies investigating the placebo effect in motor disorders such as Parkinson’s disease.41,42,43 However, Fiori and colleagues from the University of Verona recently published a study investigating changes in the cortico-spinal system during placebo induced motor performance enhancement.44 They elicited increases in force production by generating expectations about the efficacy of transcutaneous electrical nerve stimulation and used transcranial magnetic stimulation to show, that this increase in force was caused by a rise in corticospinal excitability.
Electroencephalography (EEG)
EEG is a scientifically well-grounded method based on recording voltage fluctuations from multiple electrodes placed on the scalp (non-invasive). These voltage differences represent neural brain activity with millisecond precision. This high temporal resolution allows precise tracking of parameters such as attention, emotional engagement or wakefulness. Decades of EEG research provide a solid base of knowledge about the implications of the measured data. However, only few studies so far have used EEG to look at difference in neural processing between placebo and real treatments.45,46,47
Brain activity during endurance exercise has not been investigated much because of the difficulties involved in measuring cortical parameters in sport science. During exercise large movement artifacts arise, which can negatively affect EEG signal quality.
Standardized test conditions on a bicycle ergometer, can however minimize the influence of artifacts and enable the recording of cortical parameters during exercise.48 To completely understand affective and perceptual responses during exercise and central fatigue mechanisms, it is crucial to understand the brain processes involved.49,50,51 Studies have shown, that changes in brain activity occur secondary to the metabolic changes associated with central fatigue during prolonged exercise52,53,54 and activity in the frontal cortex is associated with affective55 and perceptual49 responses to acute bouts of exercise.
Some research has been conducted on the brain level mechanisms underlying motor performance by comparing cortical activation patterns of individuals of different skill levels in specific complex sports related tasks. This is becoming a popular approach in the field of sports performance research and has been applied in disciplines such as rifle shooting,56,57 golf,58,59 karate60 and archery.61 However, only a handful of studies attempted the analysis of brain activity during endurance exercise so far. The studies which employed EEG unfortunately recorded from only few electrodes, thus limiting the informative value.62,63 Already in 1985, Nybo and Nielsen recorded EEG during exercise at 60% VO2 max in normal and hot ambient temperatures and found no changes in EEG at 18 ºC but an increase in the ratio of alpha to beta frequency at 40 °C. Unfortunately this study also recorded from only three electrode sites: left frontal, midline central and midline occipital.49 A meta-analysis on EEG responses during and after exercise showed increases in alpha, delta, theta and beta activity during and following exercise. The authors argue that understanding EEG brain activity during experimental manipulations like fatigue requires EEG responses to exercise under normal controlled conditions to be understood first.64 Bailey and colleagues examined multiple frequency bands from lateral (F7, F8, F3, F4), central (C3, C4) and parietal (P3, P4) sites during different intensities of aerobic exercise to volitional fatigue. They found global increases in theta, alpha and beta frequencies during exercise and suggest future studies to examine the association between changes in EEG and changes in cognition, emotions, and perception as well as the influence of peripheral physiology on EEG during exercise.65
Functional Near-Infrared Spectroscopy (fNIRS)
fNIRS is a functional neuroimaging method to measure brain activity through hemodynamic responses associated with neural activity. It is entirely non-invasive and is based on measuring Near-infrared (NIR) light attenuation and temporal or phasic changes.
Light in the NIR spectrum (700-900 nm) can pass unfiltered through skin, tissue, and bone, while being partially absorbed by Hemoglobin (Hb) and deoxygenated-hemoglobin (deoxy-Hb). It is the difference in the absorption spectra of deoxy-Hb and oxygenated Hb by which the relative changes in hemoglobin concentration is measured. fNIRS applies the principle of neuro-vascular coupling, known as the BOLD (BloodOxygenation-Level-Dependent) response. Neuronal activity is associated with changes in localized cerebral blood flow, but cannot measure cortical activity more than 4 cm deep and has limited spatial resolution.66
Interestingly, fNIRS is also becoming a widely used instrument for measuring muscle O2 saturation and changes in muscle hemoglobin volume. Muscle O2 saturation represents a dynamic balance between O2 supply and O2 consumption in small vessels. The role of NIRS in exercise physiology is increasing (nearly, 2004) and the results of several studies suggest that NIRS is a powerful tool for being applied successfully in sports medicine,67 as NIRS can objectively evaluate muscle oxidative metabolism and its modifications following interventions.68,69 The development of light wireless NIRS devices, operating on Bluetooth basis allow the application on freely moving subjects and enable the use during exercise protocols. Several studies have shown that these wireless NIRS devices produce nearly artifact free and reproducible data.70
fNIRS can measure changes in O2 saturation both centrally on the level of the cortex and peripherally at the level of muscles. Further, it is portable and not easily susceptible to movement artifacts. It therefore seems to be the ideal tool to research the integration of central and peripheral fatigue processes and determinants of exercise performance.71
Rating of Perceived Exertion (RPE)
Perceptual effort ratings are a key compliment to objective performance measures in order to determine the degree of physical strain including information from the periphery (muscles, joints, cardiovascular, respiratory and central nervous system). The most commonly used scaling method to quantify subjective perception of effort in sports science is the Rating of Perceived Exertion (RPE) developed by Gunnar Borg in 1970.72 It was constructed to increase linearly with exercise intensity on a cycle ergometer to fit with the linear increases in VO2 and Heart Rate (HR) observed with increasing workload.
PROPOSED MODEL
This model integrates current theories of the neurobiological control mechanisms of exercise performance and central fatigue by employing the placebo response as a mean to isolate the central component of performance enhancement. An ergogenic aid may directly influence both central and peripheral fatigue mechanisms, while also eliciting a placebo response. This placebo response may in turn influence the central regulatory feed-forward signals through expectations as well as the perception of feedback signals from the periphery. The combination of all these factorsthen determines exercise performance (Figure 1).
Figure 1. Schematic of the proposed model for the involvement of the placebo effect in athletic performance
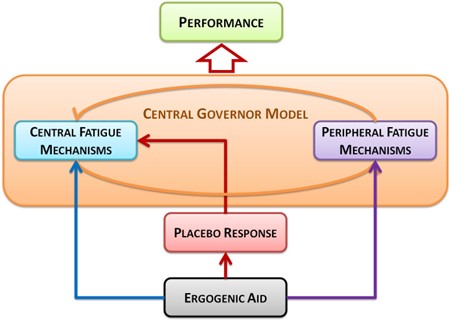
METHODS AND DESIGN
Experiment 1
The first experiment is designed to investigate the acute ergogenic effect of branched chain amino acids (BCAA) on endurance performance. The focus will be on the role of the placebo effect in enhancing endurance performance and how this effect can be modified by the mode of supplement administration. To ensure all subjects have similar expectations of the supplement, they will receive information emphasizing the acute enhancing effects of BCAAs on endurance performance. Using BCAAs as a supplement is ideal, because endurance athletes have little or no experience with the acute effect of amino acids in contrast to abundant experience with CHOs. Using BCAAs as a supplement rather than CHO (which are classically used in endurance studies) thus makes it difficult for them to guess which study group they are in (placebo vs. supplement), ensuring a solid double-blind study design. BCAAs and their placebo counterparts will be given in either food (pudding) or supplement (capsule) form. Athletes are told that they have a 50% chance of receiving BCAAs. All information regarding the intervention will be given on paper and all athletes will be verbally instructed in a standardized fashion by the same experimenter.
The Ethics Committee of the University Hospital of Tübingen has given a positive vote on the ethics proposal on January 29th, 2014 and the project is registered as a Clinical Trial at the German Registry for Clinical Trials (DRKS, DRKS00005802).
Hypotheses Experiment 1
The performance enhancing placebo response elicited by a nutritional ergogenic aid is larger when administered as food than as capsule. This is based on the assumption that stimulus salience (food>capsule) has a larger influence on the placebo response than stimulus conditioning (supplement>capsule). We thus hypothesize, that the food group will show a larger increase in performance between baseline time trial (TTb) and intervention time trial (TTi).
Methods Experiment 1
Using a 2(food vs. capsule)×2(supplement vs. placebo) factorial analysis of variance with repeated measures with an effect size of 0.25, a power of 0.80 and alpha of 0.05, 30 athletes have to be included in the study (as calculated with the software G Power). Thus, 30 ambitious male bicyclists and triathletes between the age of 18 and 40 will be recruited fulfilling the following criteria: 3-5 bicycle training sessions per week during season and regular participation in competitions (mountain bike, triathlon, road racing). Exclusion criteria were serious illness, use of medication during the time of the study and training pause in the 4 weeks immediately prior to the study. Each participant will be measured on 4 days at the performance diagnostics laboratory at the Department of Sports Medicine in Tübingen, Germany.
A 2×2 randomized double-blind repeated measures design with a no-treatment control group will be used with the factors intervention (placebo vs. supplement) and form (food vs. capsule) and motor performance measures (objective and subjective) as dependent variables. Athletes will be randomly assigned to one of 5 groups: Food supplement (FS) (N=6), food placebo (FP) (N=6), capsule supplement (CS) (N=6), capsule placebo (CP) (N=6) or no-treatment control (C) (N=6). The no-treatment group controls for training and learning effects. All athletes are subjected to 4 test sessions. During the first visit they are provided with a detailed informed consent form. Once they consent to participate in the study, they undergo a thorough examination by an attending medical doctor, who then admits them to participate in the study. Once all formal requirements are met, a performance test is performed to assess current maximal aerobic capacity (VO2 max), Lactate Threshold (LT) and Individual Anaerobic Threshold (IAT) using a stepwise test protocol (40 W/ 40 W/ 3 min) on a bicycle ergometer. This protocol begins at a work rate of 40 Watt increasing by 40 Watt at regular 3-minute intervals. The protocol goes on until subjects can no longer maintain cadence above 65 rpm or voluntarily stop due to exhaustion. At the end of each interval blood samples are taken from the right ear lobe to determine the lactate concentration at each workload. Simultaneously inhaled and exhaled air is measured via spiro-ergometry to determine oxygen consumption (VO2), carbon dioxide production (VCO2) and total ventilation (also called cardio pulmonary exercise test or CPET).
The next session serves as a test time trial (TTt), where participants are familiarized with the isokinetic SRM bicycle ergometer (SRM GmbH) in a 45 min practice session consisting of 10 min warm-up, 30 min time trial (TTp) and 5 min cool-down. The SRM is a practical tool to measure physical condition with predefined constant cadence (here: 95 rpm) and the cyclist himself determines his power output. This allows for an accurate measurement of endurance capacity in relation to a specific duration. At the end of this session, participants are expected to be familiar with the testing procedure.
During the third session the 45 min baseline performance time trial (TTb) takes place, during which the athletes are instructed to attempt to cycle for maximal average power output over time at a constant cadence of 95 rpm. The time trial is preceded by a 10 min warm-up and followed by a 5 min cool-down at variable rpm and fixed work load (1.5*bodyweight in kg).
In the 4th session the intervention with the ergogenic supplement takes place (TTi). TTi is scheduled exactly 7 days later at the same time of day as TTb (8 am or 10 am), in order to minimize influences of natural performance fluctuations. Thirty minutes prior to the time trial at TTi athletes receive their respective intervention (FS, FP, CS, CP, C), depending on which experimental group they were assigned to. The athletes have to come fasted (12 h no food and caffeine) on both test days (TTb and TTi) to ensure equal absorption rates of the supplement and control for external variables such as performance influences of caffeine and CHOs.
During both TTb and TTi performance is measured along with physiological measurements before, during and after the test. During the trial, blood samples (20 µl) are collected from the ear lobe in 5 min intervals for lactate diagnostics and the pulse is measured via a chest strap heart rate sensor.
To control for potential covariates, athletes will also report subjective performance assessments and perceived level of exertion (RPE)73 in addition to general personal information (e.g. training frequency, performance in the past season, position on the team, demographic data etc.) and four genuine German sports performance motivation questionnaires (AMS: Achievement Motives Scale-sports; HOSP: Action orientation in sports; SOQ: Sport Orientation Questionnaire; VKS: Volitional components is sports).74
Experiment 2
The second experiment aims to unravel the central processes underlying enhanced athletic performance and how this information ties in with current models of fatigue. To research this underlying mechanism we initiate performance changes via a placebo ergogenic aid to isolate the central component of performance enhancement and then measure the underlying neurobiological processes using Electroencephalography (EEG).
Hypotheses Experiment 2
To our knowledge, no study has examined the central mechanisms underlying placebo induced performance enhancement by nutritional ergogenic aids. However, we assume that it is associated with changes in brain activity measurable by EEG. The data will therefore be analyzed in an exploratory fashion on the one hand and hypothesis driven on the other hand. We will attempt to replicate the findings of the metaanalysis by Crabbe and Dishman, suggesting that EEG responses during and after exercise show increases in alpha, delta, theta and beta activity.64 We further aim to analyze the association between changes in EEG and changes in fatigue perception and the influence of peripheral physiology during exercise as suggested by Bailey and colleagues.65
Methods Experiment 2
As soon as Experiment 1 is evaluated, Experiment 2 will employ a similar study protocol as in Experiment 1, however, with a different group of athletes. Depending on the outcome of the first experiment, the mode of administration (food vs. capsule) that showed the largest performance enhancement will be used in the second experiment. A repeated measures design will be used with intervention (control vs. placebo) as independent variable and central and peripheral performance measures (objective and subjective) as dependent variables. In order to achieve a medium effect size of 0.25 (power=0.80, α=0.05) using a one factorial repeated measures analysis of variance (placebo vs. control), 30 athletes have to be included in the experiment. Athletes will be randomly assigned to one of 2 groups: Placebo (P) (N=15) or no treatment (control) (N=15). However, athletes in the placebo group will be told that they are given an ergogenic supplement that will enhance their endurance performance. EEG will be recorded from 64 electrodes according to the international 10-20 system before, during and after the 45 minute time trials at TTb and TTi.
Experiment 3
A third experiment will employ functional Near-Infrared Spectroscopy (fNIRS), which is a non-invasive measurement of the oxygen content of hemoglobin associated with neural activity. fNIRS is portable and even wireless instrumentation is available, enabling measurements in freely moving subjects. Based on the outcomes of the first two experiments, this third experiment is designed to enhance the understanding of the mechanism limiting and determining motor performance by integrating and regulating central and peripheral fatigue processes.
This experiment will further enable the specific investigation of the feed forward and feedback signaling pathways involved in performance and fatigue perception, because fNIRS can be used to measure both brain activity and muscle metabolism simultaneously.
The hypotheses for this study are based on the results of experiment 1 and 2, thus no exact information on the statistical analysis of this fNIRS experiment can be given at this time. However, the experimental procedure will be similar to that of experiment 2 and we expect to include further 30 athletes in this experiment.
CONCLUSION
This study protocol approaches a cross sectional area of research between exercise science, neuroscience and behavioural sciences. Most of the institutes interested in the combination of these research areas, however, do not go beyond behavioural data due to a lack of neuroimaging equipment. Not only does this study clarify the issue of the influence of the mode of administration of an ergogenic aid on the degree of performance change. It will also show us, which brain mechanisms mediate this enhancement independent of the physiological effect any ergogenic aid may have. Data from the present study will thus enhance our understanding of the neurobiological basis of central factors limiting and enhancing physical performance and further our knowledge of the role of the placebo response and thus the psychosocial context on exercise performance. Furthermore, understanding the principle mechanisms underlying enhanced motor performance is relevant for the prevention and treatment of diseases which may be caused by a lack of physical activity (e.g. obesity, diabetes, heart disease, high blood pressure, etc.) or which may cause a decrease in the ability to be physically active (Morbus Parkinson, depression, etc.). Further this knowledge can be practically applied in the treatment and interaction with athletes in the context of sports medicine, physical therapy and nutritional interventions. Finally, investigating the placebo effect in the context of motor performance elicited by the administration of a nutritional ergogenic aid has implications not only for sports and the development of training strategies,26,75 but also for the direction of future performance research and the controversial topic of (placebo) doping in sports,76 raising not only ethical but also legal issues.
COMPETING INTERESTS
The authors declare that they have no competing interests.
ACKNOWLEDGEMENT
We acknowledge support by Deutsche Forschungsgemeinschaft (WE 5658/2-1) and the Open Access Publishing Fund of the University of Tübingen.
AUTHORS CONTRIBUTIONS
EB: designed the study, conceptualized methods and research protocol, wrote the paper, will carry out the study, acquire and analyze the data. PE, KW: will support analysis of the data and paper writing. PE, AN: responsible for conception, study design and will support the preparation and funding of the study. PS: supported development of endurance protocol, conception of physiological measurements, will support data acquisition and study preparation. KW: advised on the study protocol, supported the writing process and revised the article. All authors reviewed and approved the final draft of the paper.