INTRODUCTION
Soccer is an intermittent sport, made up of periods of low intensity activity such as running and short high intensity movements such as sprinting and jumping.1 These repetitive activities place the performer at considerable risk of injury, particularly to the Achilles Tendon (AT).2,3,4
Susceptibility to AT pain and injury has been reduced via the use of commercially available heel inserts.5,6,7 Similarly, these devices have been used successfully in the treatment of AT injury.8 The mechanism behind the reduction in pain and injury is unclear, although one theory suggests that heel inserts change the orientation of the foot, raising the heel relative to the forefoot.9,10 Such orientation is thought to limit the calcaneal friction11 and lower the maximum dorsi-flexion angle during the mid-stance phase of gait. This is believed to lessen the eccentric force and strain applied to the tendon, used to control the downward movement of the centre of mass.9
To quantify the mechanical change that occurs in response to a heel lift intervention, authors have measured external kinematic data.12,13 Others have estimated internal load14,15 although findings are inconsistent. Where differences in load have been shown,15 the magnitude of the heel lift required is greater (18 mm) than the lift found to reduce pain and injury (2.5-15 mm).7,8,10 Consequently, the mechanism behind the successful application of smaller heel inserts is still unclear.
One potential reason for the lack of supportive findings may relate to the measurements being used to quantify internal load. During running, it is assumed that between 85-100% of the net force generated during dorsi-flexion is from the triceps surae muscle group. This force is then applied via the AT to control the body’s downwards movement.16,17 This measurement has therefore been used as an indicator of AT force.11 Such measurement however, does not directly estimate AT force. Such calculation requires knowledge of both the force and the moment arm about the joint axis of rotation. Dixon18 presented a method that provides a reliable, subject specific estimate of the forces occurring in the AT. However, the accuracy of estimations using this approach has been limited by the use of Two-Dimensional (2D) estimates of both joint moment and AT moment arm. Another problem is that some studies have used barefoot trials with the heel inserts strapped to the foot.14,19 Due to participants being unaccustomed to such conditions, this may cause them to exhibit an altered running gait which may have resulted in the lack of significant findings observed.20 Use of a soccer boot may therefore result in a more typical running strategy in those who are familiar with wearing the footwear. Alternatively, it is possible that the rate at which the AT structure is loaded rather than the peak force applied may be indicative of the mechanism behind reduced pain and injury.14 Individual mechanical differences in running gait are also an inherent source of variance in running patterns and may influence the user’s response to the heel insert intervention. Consequently, some athletes have experience significant alterations in estimates of loading while others did not.11,14 Such a reason, may explain literature findings that describes no effect of a heel insert intervention on injury risk.21 As a consequence, looking at changes in AT load using pooled data may disguise the response of some individuals to the intervention.
The aim of the present investigation is to address the previous studies limitations, to assess the influence of a commercially available heel insert on peak plantar flexion moment and estimated AT force experienced by soccer players. Likewise, the relative importance of the average loading rate of these measurements is also investigated. It is hypothesised that the magnitude of peak plantar flexion moment and peak AT force, and the average rate of loading of these measurements, will be significantly reduced with the inclusion of the heel insert into a soccer boot when running at sub-maximal velocity.
METHOD
Nine male amateur soccer players (83.4 (±5.8 kg), 23 years (±3.7), Achilles tendon radius 19.13 cm (±2.3), ankle width 0.072 cm (±0.005), forefoot width 0.10 cm (±0.005), size 10 feet) participated in this research investigation. All participants were heel-toe runners as indicated by distinct, double peaked force-time histories.22 All participants regularly participated in soccer and had recent experience of playing on a third generation artificial surface. Each participant was pain and injury-free for the three months prior to data collection. All participants provided written consent in accordance with the University Human Ethics Committee (UHEC).
Fifteen metres of third generation artificial shock pad (Arpro® Expanded polypropylene, 24 mm±0.5 mm thick, Brock International) with a measured density of 65 g and a mechanical hardness of 1254.3 N (S.D. 48.5 N), was laid across a concrete laboratory floor. Placed upon the shock pad was a third generation turf of similar length (Astroplay MXS 40, Lano sports, Herelbeke, Belgium) and 10 kg·m2 of sand mixed with 8 kg·m2 of rubber crumb (5:4 ratio of sand to rubber). This was distributed as recommended by the manufacturer. An AMTI force plate (960 Hz) was positioned underneath the surface, approximately in the centre of the surface in both width and length. This was represented by a square marked by tape on the surface.
Participants performed 10 running trials upon the artificial turf surface as this is sufficient to obtain stable mean data for an individual.23 The trials were performed whilst wearing a soccer boot with a moulded stud configuration (Adidas, Copa Mundial) as the control condition. For the experimental condition, a commercially available 10 mm Sorbothane heel insert (Sorbothane Shock Stopper, Sorbopro, Layland, Lancashire, UK) was placed inside each shoe. These conditions were tested for each participant in a randomised but balanced order.
The participants were asked to run the length of the 15 m artificial turf surface at a speed of 3.81 m.s-1 (±5%), monitored by photosensitive timing gates positioned one meter either side of the force plate. Sufficient time was given for the participants to establish a normal running style that ensured that they placed their right foot within the marked area without changing their normal stride pattern; they then continued their run to the end of the artificial turf surface. Participants adjusted their start position to ensure they were able to hit the centre of the marked area. All trials were monitored by the researcher and any trial that was not performed as directed or which were not at the correct speed were subsequently repeated.
To calculate joint moments, each participant wore retro-reflective markers on the right side of the body (Figure 1). An eight camera (Pulnix, TM-6703 progressive scan, 120 Hz) automatic tracking system (Vicon, Motus version 6.1, Englewood, CO, USA) was used to capture these markers and calculate their coordinates via the application of Direction Linear Transformation (DLT). These markers were then used to generate local reference co-ordinate systems based on the methods described by Soutas-Little, et al.24 Quintic splines25 were fitted to the raw coordinates to obtain smooth continuous time histories for first and second derivatives. Acceleration data were calculated by the first central difference method.
The calculation of Three-Dimensional (3D) moments about the ankle joint used inverse dynamics and required the measurements of ankle joint and forefoot width to allow estimation of the ankle joint centre and the distal point of the foot segment. Ankle width was calculated by measuring the distance from the lateral malleolus to the medial malleolus with a calliper; forefoot width was measured as the distance between the first and fifth metatarsal at the widest location. This information was entered into the motion analysis software and joint centre and distal end points were calculated. When determining forefoot width, shoe material thickness was regarded as negligible. The inverse dynamics calculation also required additional knowledge of foot moment of inertia, mass and centre of mass. The foot mass and centre of mass were calculated using adult male cadaver data from Clauser, et al26 and moment of inertia data using the data provided by Whitsett.27
Figure 1. Images identifying marker placements used in the moment and moment arm calculation
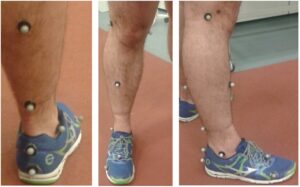
To collect kinetic data, specifically force (Fx, Fy, and Fz), centre of pressure (ax, ay) and free moment (Fm), a force plate (AMTI, Newton, MA, USA) was used. The synchronous force and smoothed coordinate data were transferred from the Vicon Motus software into a Matlab program (Matlab, 7.0.4, The Maths Works, USA). Within the Matlab program, a code was written that interpolated the 960 Hz kinetic data to 120 Hz.
The calculation of three-dimensional moments occurring during plantar flexion was performed using code based on previously published methods.28,29 During the movements, the conventions of the calculated muscle moments were that a negative moment represented a resistance to extension of the segment. Three-dimensional AT force was calculated by adopting a similar technique to that developed previously.14,19 However, in the present study, a three-dimensional moment arm was calculated as the perpendicular distance between the ankle joint centre and the line of the AT represented by the two markers on the posterior aspect of the shank.
To account for the influence of the skin thickness that surrounds the tendon sheath and the radius of the external marker on the calculation of moment arm length, the radius of the marker and the skin covering the AT, was removed from the moment arm length prior to the calculation of AT force. To calculate the skin thickness, the radius of the AT width at approximately 5 mm from the AT insertion point was measured with a calliper for each subject. This was scaled using the skin thickness-AT radius ratio reported previously as 3.9 mm skin thickness when the AT radius was 7 mm.18 Peak plantar flexion moment and AT force were then determined from the calculated moment data. Average loading rates for each of these values were also calculated by dividing the peak moment and AT force by the time over which it had occurred.
For all participants, the mean and standard deviation of the 10 trials per condition was calculated and statistically compared using a paired t-test. Normality of data distribution was tested by determining skewness and kurtosis statistics. Individual participant data was also separately analysed by paired t-tests, comparing the corresponding trial data between the two conditions.18 The alpha level was set at 0.05 for all statistical tests which were performed using SPSS (IBM SPSS 21, New York, NY, USA).
RESULTS
The use of a 10 mm heel insert did not significantly reduce the estimated loading on the AT as indicated by the measurements of peak plantar flexion moment and peak AT Force. Similarly there were no significant differences observed for the measurement of average plantar flexion moment or average AT loading rates (Table 1).
Table 1. Mean±SD for plantar flexion moment and Achilles tendon force and corresponding loading rate data obtained
whilst running in soccer boots with and without a heel insert
|
Control
|
Heel insert |
P
|
Peak plantar flexion moment (N) |
196.9±73.5
|
192.5±53.3
|
0.68
|
Average plantar flexion moment loading rate (Nm.s-1) |
1612.2±526.6 |
1617.4±795.7 |
0.97
|
Achilles tendon force (BW) |
6.6±3.0
|
7.3±4.0
|
0.25
|
Average Achilles tendon force loading rate (N.s-1) |
255715.5±109306.8
|
259396.3±152438.3
|
0.92
|
Individual data analysis was performed by conducting separate paired t-tests for each participant and comparing between conditions using the corresponding trial data. This analysis indicated that there were significant individual responses to the heel insert. Some participants demonstrated a significant increase in loading and loading rate whilst others showed significant reductions; other participants showed no significant change in these measurements (Figures 2-5).
Figure 2. Means and standard deviations for each individual participant for the measurement of plantar flexion moment collected whilst running with the control (no insert) and experimental (insert) conditions
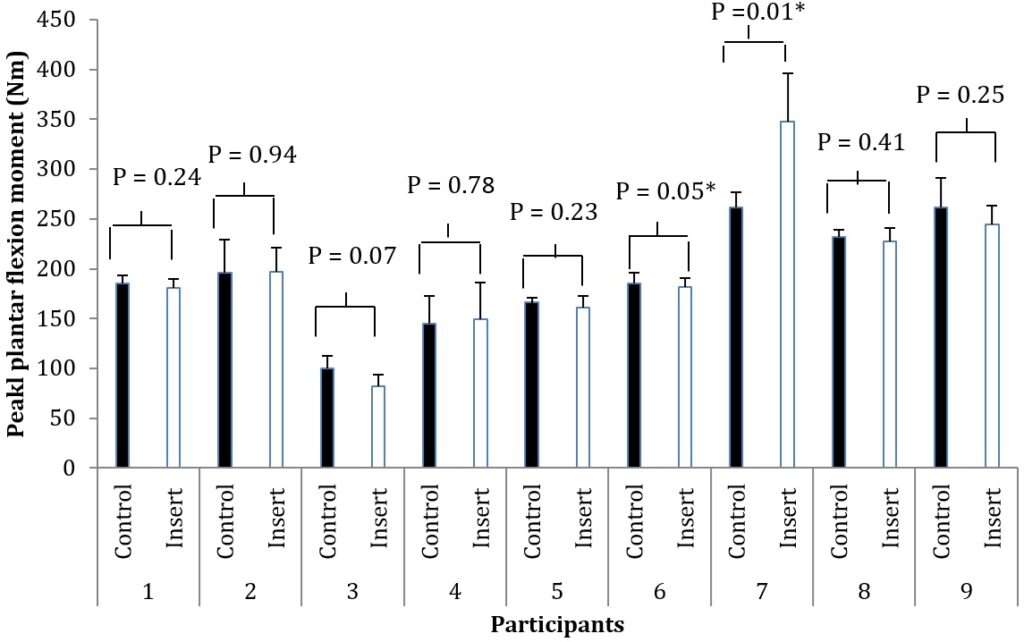
*denotes a significant difference at the p<0.05 level.
Figure 3. Means and standard deviations for each individual participant for the measurement of average plantar flexion moment loading rate collected whilst running with the control (no insert) and experimental (insert) conditions.
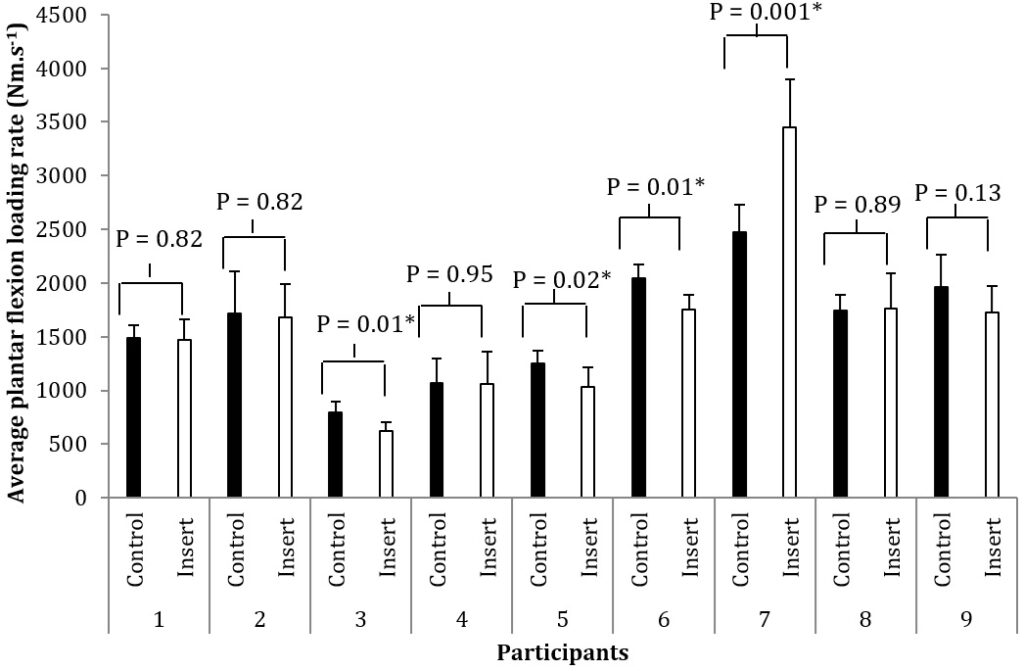
*denotes a significant difference at the p<0.05 level.
Figure 4. Means and standard deviations for each individual participant for the measurement of peak Achilles tendon force (measured in body weights, BW) collected whilst running with the control (no insert) and experimental (insert) conditions.
*denotes a significant difference at the p<0.05 level.
Figure 5. Means and standard deviations for each individual participant for the measurement of average Achilles tendon force loading rate collected whilst running with the control (no insert) and experimental (insert) conditions.
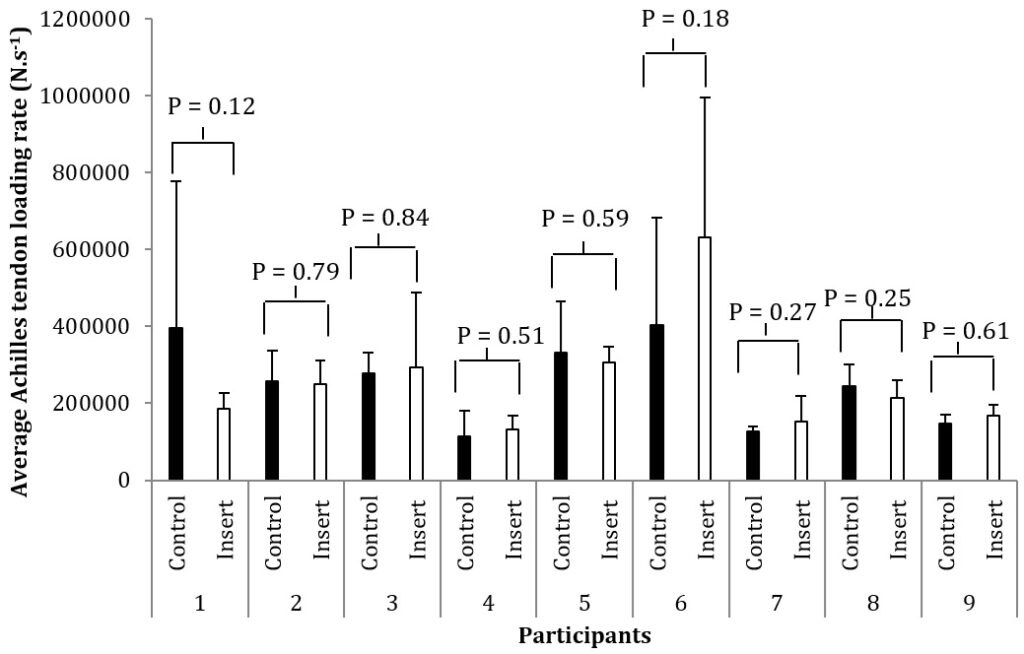
DISCUSSION
The aim of this study was to address the limitations of previous studies to better understand the mechanism behind injury reductions with the use of heel inserts. Despite the use of three-dimensional moments and moment arm to calculate AT force, and the use of footwear, the data failed to support the hypotheses that peak plantar-flexion moment and peak AT force would be significantly reduced. Similarly, the rate at which the AT was loaded was also not significantly reduced when wearing the insert.
When looking at the individual data, a large range of peak moment and AT forces magnitudes was shown between participants. Such variation is unlikely due to the methodological approach since the approach has been shown to be reliable.18 The estimation of AT force in the current study produced values of approximately 5 and 6 times the body weight of the participants, which is within the magnitudes reported by Komi30 when in vivo AT forces were measured. Calculate moment arm lengths of between 30-40 mm19 are also comparable to those calculated by Magnetic Resonance Imaging (MRI).31,32 These are slightly longer than found previously15 although morphology differences in the participant groups could explain this. The approach did not account for any opposing (antagonistic) contribution of muscles such as the tibialis anterior which may contribute to a small underestimation of the loading.15 Likewise, failure to account for any additional assistance from the other plantar flexors to the net muscle moment may have influenced the magnitudes observed.15 However, the contribution of these muscles have been described as ‘trivial’ and activation profiles have been shown to be similar with and without larger inserts being applied.15 Study findings are also unlikely to have been influenced by differences in running velocity, since consistency in velocity was assured via the use of timing gates. All participants were also identified as heeltoe runners which meant differences in landing style are also unlikely to have influenced findings.
An alternative explanation for these findings may be that the response to the heel insert intervention is highly individual.11,14,19 The paired t-tests performed for each participant separately showed that despite the similar landing strategy, two of the nine participants exhibited significant differences in peak plantar flexion moment. One of these participants exhibited greater peak moment and the other a decrease with the heel insert condition. The participant who experienced an increased moment also experienced a corresponding increase in AT force, suggesting the increased moment magnitude contributed to this rise in AT force. On the other hand, the participant who experienced a reduction in moment experienced no change in AT load. A shorter moment arm when running with the heel insert could explain this finding, resulting in comparable overall force. Further still, two participants showed significant reductions in peak AT forces with the heel insert, without a change in the moment magnitude, which again highlights the influence a change in moment arm can have on AT force. Various individual responses were also shown in the calculated loading rate for joint moments but not AT loading rate which may relate to reduced statistical power for this measurement. Such individualized responses are supportive of other research on heel inserts11,14,19 and indicate the mechanism behind injury reduction is varied. Whilst the participants in the current investigation were all injury free at the time of testing, individuals with reduced peak forces would therefore respond more favourably to the intervention when injured. By contrast, given sufficient repetition, those with increased loads may experience worsening of their symptoms if injured. Treatments using a heel insert should therefore be performed on an individualised basis and regular monitoring of the clinical responses (i.e. symptoms getting worse) is needed.14
It has been suggested that those with highest AT force under the no heel insert condition, experience the significant reductions with heel-insert when those with lower forces do not.14 This trend was however not supported in the current investigation. Whilst heel-toe landing strategy was consistent, the individual response may relate to specific physiological differences such as flexibility at both the ankle and knee joint due to the bi-articular nature of the gastrocnemius muscle. Future studies investigating the protective and rehabilitative benefits of heel inserts need better understanding of these differences to investigate the causative factors for individual response.
It is also important to acknowledge that the aetiology of AT injury has been related to a change in calcaneal friction rather than force magnitude. Reinschmidt and Nigg11 postulated that reduced inflammation may occur when the calcaneus is lifted with respect to the tibia. Such suggestion may however only explain the treatment benefits for those with insertional Achilles tendonopathy.11 It has also been found that the amount of strain of the muscle resulting from the applied force is significantly reduced with a heel insert. This suggests that rather than reducing overall force, the heel insert lowers injury risk by reducing that amount of lengthening the tendon undergoes.12,15 Another problem that arises from the use of peak plantar flexion moment and AT force is that the magnitude only refers to axial loads or a stretching of the AT.11 The change in heel height may change non-axial loads, such as shear and/or bending. This may be affected by the amount of pronation that occurs which has been shown to be influenced by heel height.33,34
In conclusion, the investigation reported no significant change in group data when a 10 mm heel insert in used, suggesting that measures of Achilles tendon loading could not indicate the mechanism behind reduced injury incidences. Therefore, these results do not support the original hypothesis. However, single subject analysis suggest that the response is individual and that reduced loading is a suggested mechanism behind the reduction of injury risk and successful treatment for some. Caution is recommended however, since significant increases in peak AT forces were shown for some individuals, suggesting that for these individuals, an increased risk of injury or worsening of symptoms can occur with the application of a heel lift intervention.
CONFLICTS OF INTEREST
The authors declare that they have no conflicts of interest.