The repetitive nature of endurance running1 and the transference of energy generated on impact with the ground2,3 is entwined with reported high incidences of lower extremity injuries.4,5,6 Previous reports suggest incident rates of those embarking on training for distance events are between 2.5-12.1 per 1,000 h6 with specific reports regarding those training for a marathon equating to >70% of participants.7 Such odds, makes training for events like the marathon a particularly risky business and there seems to be no distinguishable preference to individual performance status.8
As such the injured runner faces the predicament of a cessation in training, the onset of an initial negative psychological/emotional response(s),9 followed by differing degrees of physiological and subsequent performance detraining effects.10 Justifiably, such emotional r sponses are based on the substantiated and well documented understanding that physiological function decreases after as little as 3 weeks cessation11,12 due primarily to cardiovascular system changes.13,14,15 However, the differentiation between cessation of training and a reduction in training load (tapering response) in terms of physiological and performance response is critical. Tapering involving the reduction of training load, is associated with performance improvement.15 This is due to reductions in physiological and psychological stress which increase peripheral fatigue. As such, any intervention that can stimulate a physiological response close to normal training conditions in an injured runner, while not hampering the recovery process, must be seen as positive to an otherwise quite traumatic situation.16 It has been suggested that deep water exercise17,18,19 or other non-impact exercise18 offers sufficient stimulus to preserve prior fitness levels amongst healthy participants. However, no assessment of the impact of such an intervention on running dynamics has been performed. More recently, water immersed treadmill exercise has become popular as a low risk, more specific mode of training for endurance runners. Aquatic treadmill (ATM) running has been shown to unload vertical forces by an estimated 70% compared to Land Treadmills (LTM) due to decreased velocity at impact, hydrostatic pressure and the buoyancy effect of water immersion.20 Importantly, it is suggested that the anatomical structures that benefit from the reduced load of ATM training are the hip, knee and foot joints along with the passive structures of the lower limbs. These normally take the strain in land based running21,22 and are most at risk of injury amongst endurance runners.6 Therefore, it is feasible to suggest that ATM running could be used as a means of rehabilitating muscle movement patterns without the risk of re-occurring injuries. Furthermore, the understanding that ATM compared to LTM running can result in greater metabolic costs23,24,25,26 suggests it could be used as a means for maintaining specific cardiovascular fitness and therefore performance during periods of injury amongst runners. However, such suppositions are unsubstantiated for an injured athlete(s) and it is unknown how such periods of training might effect normal running dynamics on return to land based training. Nevertheless, to be of real benefit to athletes trying to maintain fitness during normal training cessation there would have to be similar physiological demands of exercise without incurring the same risks as land based training.
Therefore, the purpose of this case report is to establish the efficacy of waist depth ATM running throughout a period (28 days) of injury to the lower leg, where normal land based running was not possible. As such the emphasis was to assess and compare post-injury LTM running spatio-temporal parameters and the subsequent physiological responses with pre- injury measures at a sub-maximal running velocity.
METHODS
Participant
One nationally competitive masters distance runner (height 172 cm; body weight (land) 58.4 kg; body weight (water, waist depth) 28.4 kg; land based BMI 19.74; V̇O2max 68 ml·kg-1·min-1; HRmax 168 bpm) with a 5,000 m treadmill time trial performance of 14 min 58 s prior to injury and who had prior experience of LTM and ATM running, participated in this case study after giving written consent in accordance with the University Human Ethics Committee. The participant was part of a separate study investigating running dynamics during LTM and ATM20 but presented themselves with an accute injury soon after completion of the initial study. The injury was diagnosed by a qualified phsyiotherapist as a Grade 2 (2nd degree moderate) calf strain with a loss of strength and inability to continue any form of land based running or similar activity. Treatment of the diagnosed injury involved 2 days of complete rest including application of the RICE principle, a return to exercise based on pain free experience, and self-application of massage as directed by physiotherapist.27,28
Training
Training prescription was not controlled during the case report period but the participant was asked to manage training in relation to a non-painful intensity and/or volume during the injury rehabilitation period. In this respect all training performed was of a low intensity and of a continuous nature. An electronic training diary (RubiTrack3, version 3.4.7) was kept prior to and throughout the whole period of the case study and training load (a measure of training intensity and volume) is expressed as Training Impulse (TRIMP).29,30
TRIMP=Exercise Duration (mins)×[(HRex-HRrest)/(HRmax-HRrest)] (Eq.1)
Where, HRex is the mean HR for the training session; HRrest is the participants resting HR; HRmax is the participants maximum HR.
Figure 1 highlights the participant’s weekly training load throughout the period of the case study. The training load values during these weeks translated to weekly distances: 119.0; 74.2; 83.6; 77.9; 172.9; and 184.7 km with a corresponding average heart rate of 125; 121; 124; 121; and 130 bpm, respectively. This data indicates that the type of training undertaken throughout the whole period was in the low intensity, high volume category.
Figure 1. Participants Training Load (TRIMP) throughout the Period of the Case Study
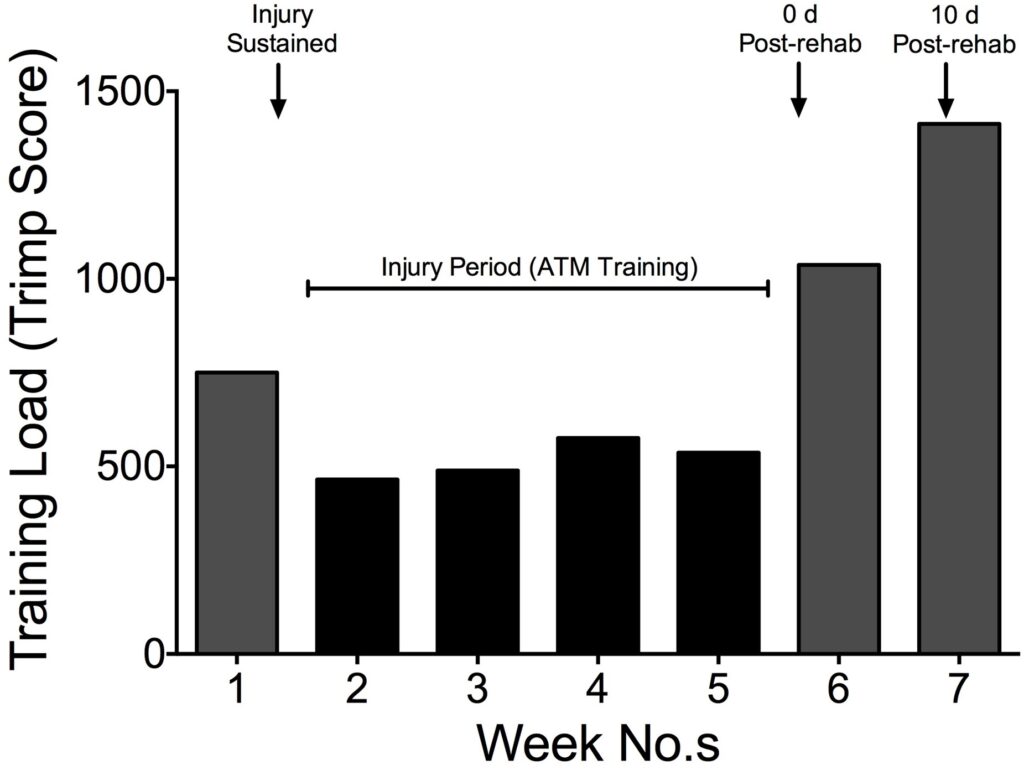
Procedures and Measurements
On arrival at the laboratory the participant was measured in order to determine the water depth for ATM (height from floor to anterior superior iliac (cm) which was kept constant throughout trials) and weighed (dry land body weight and body weight (kg) at the predetermined immersion depth).
Following this the participant was fitted with the wireless, tri-axial accelerometer (Emerald, APDM, OR, USA), used to measure accelerations (reported accuracy 0.0012 m·s2 ·√Hz-121) of the distal anterolateral aspect of the right tibia, selected to minimize soft tissue oscillations during impact21 and not infers with gait patterns during ATM running. The accelerometer was packed tightly into a waterproof sport armband housing (h2 oaudio, San Diago, CA, USA) and secured tightly. In addition to this the participant was connected to an automated portable gas analyzer (K42b, Cosmed, Italy) that sampled expired air on a breath-by-breath basis; an ANT+ heart rate monitor – Run strap plus transciever (Garmin Forerunner 620, USA).
Following a warm-up and familiarisation period of 5 min at <2·78 m·s-1 prior to both conditions, the participant ran at the required speed of the trial for 2 min in order to eliminate any differences between oxygen kinetics that may occur at the onset of exercise due to water immersion.31 The experimental protocol consisted of the participant running at 2.83 m·s-1 over a 15 min period, on both LTM (TechnoGym, Italy) and ATM (O’Leary Engineering, New Zealand), and separated by a 15 min recovery period. The ATM water depth was set to anterio supra iliac (105 cm) with water temperature maintained at 21 °C throughout all trials. It was felt that the selected water depth offered the least disturbance to arm swing whilst running and has previously been shown to balance the effects of increased resistance with increased buoyancy in terms of work done comparable to LTM.25 Each treadmill was calibrated prior to each trial, to the same speed (Eq.1) by using a magnetic switch that was triggered with each belt revolution.
Treadmill speed (km·h-1)=((((Treadmill Belt length (m)×revs·min-1)/1)×60)/1000) (Eq.2)
The different trials were distinguished by the duration of time (Days) in relation to the participant rehabilitation status, and included: non-injured (Day 0); complete rehabilitation, post injury (Day 28); post injury (Day 38). Each condition (LTM and ATM), within trial, was performed in the same order to enable comparison between pre-injury and post injury values.
Outcome Measures
All variables were logged over the full 15 min period. Accelerometery data (128 Hz) was processed using MATLAB R2014a, analyzed for total (XYZ) accelerations (m·s-2) allowing identification of the precise times of foot contact, peak impact and toe-off for each stride (Figure 2).
Figure 2. Accelerometer output for total accelerations (XYZ-planes) versus time (s) at the foot for the LTM (A) and ATM (B) conditions
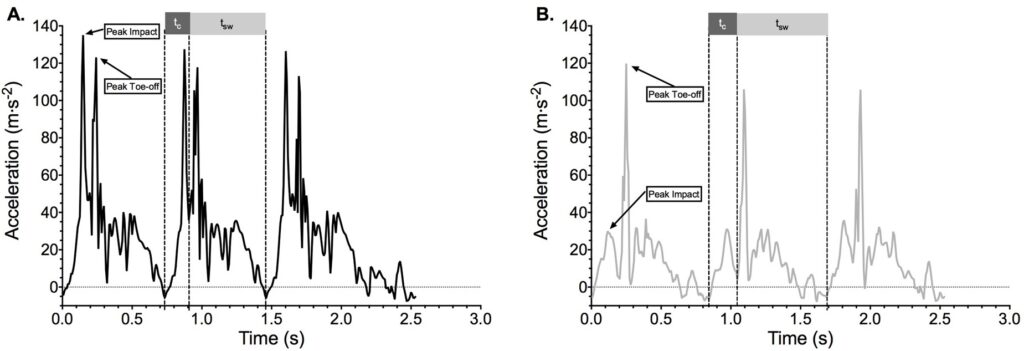
Ground contact (tc) and swing time (tsw) are highlighted along with peak impact and peak toe-off.
From these accelerometric outputs the following variables were measured or calculated for the complete 15 min period: a) ground contact time (tc), defined as the time (s) from initial foot contact to the point of toe-off5; b) swing time (tsw) defined as the time (s) from toe-off to intitialinitial ground contact of consecutive footfalls of the same foot5; c) stride duration defined as the time (s) taken for consecutive peak impacts of the same foot; d) stride frequency (Sfreq) defined as the number of strides taken per second (Hz); e) stride length (m) was calculated by dividing treadmill speed (2.83 m·s-1) by Sfreq(Hz).
Expired respiratory gases collected breath-by-breath enabled the calculation (CPET suite, version 10.0e, Cosmed, Italy) of: the body’s rate of oxygen utilisation (V̇O2, expressed ml·kg-1·min-1); the ratio of the carbon dioxide produced to oxygen used (Respiratory Exchange Ratio, (RER)); the amount of air expired from the lungs during a minute (minute ventilation (VE, L·min-1); and breathing frequency (Rf, breaths per minute).
Spatio-temporal and physiological data were analyzed collectively as a means to determine efficiency of the two conditions via the oxygen consumed per stride (V̇O2 per stride, ml).32
Data Analysis
Data generated for all descriptive dependent variables was collected over the complete 15 min period and analyzed over 5 min epochs for physiological and spatio-temporal variables. Data for all variables is expressed as the mean over the specific epochs unless otherwise stated.
RESULTS
Running Dynamics
Data analysis for each 5 min epoch showed no changes over time within and between each trial for basic measures of running dynamics for either LTM or ATM. Therefore, subsequent analysis is presented as an overall comparison between trials (Day 0, 28, and 38) and conditions (LTM vs. ATM).
Stride duration (Figures 3A and 3B) was considerably different between LTM and ATM from the onset (0.737±0.003 vs. 0.884±0.012 s, respectively) of the case report, but this difference was reduced following 28 d of ATM training (0.778±0.007 vs. 0.840±0.013). Interestingly, the analysis at 10 d post-injury (Day 38) shows a reduction in LTM stride duration but an increase in ATM stride duration. Percentage differences equated to 120.0±1.2, 108.0±1.8, and 122.8±0.6%, for the three time points respectively. Key components of gait analysis (tc and tsw), presents a slight parabola effect for LTM (0.205±0.007, 0.230±0.002, and 0.209±0.007 s; 0.528±0.008, 0.550±0.002, and 0.544±0.008) and an inverse parabola for ATM (0.211±0.009, 0.176±0.015, and 0.208±0.004; 0.689±0.010, 0.677±0.010, and 0.736±0.012) for Day 0, 28, 38 time points for both tc and tsw, respectively (Figures 3C-3F).
Figure 3. Mean±SD, for specific components of stride presented in time (s) and separated by
condition (LTM and ATM) over each trial specific to the injury recovery time point reference
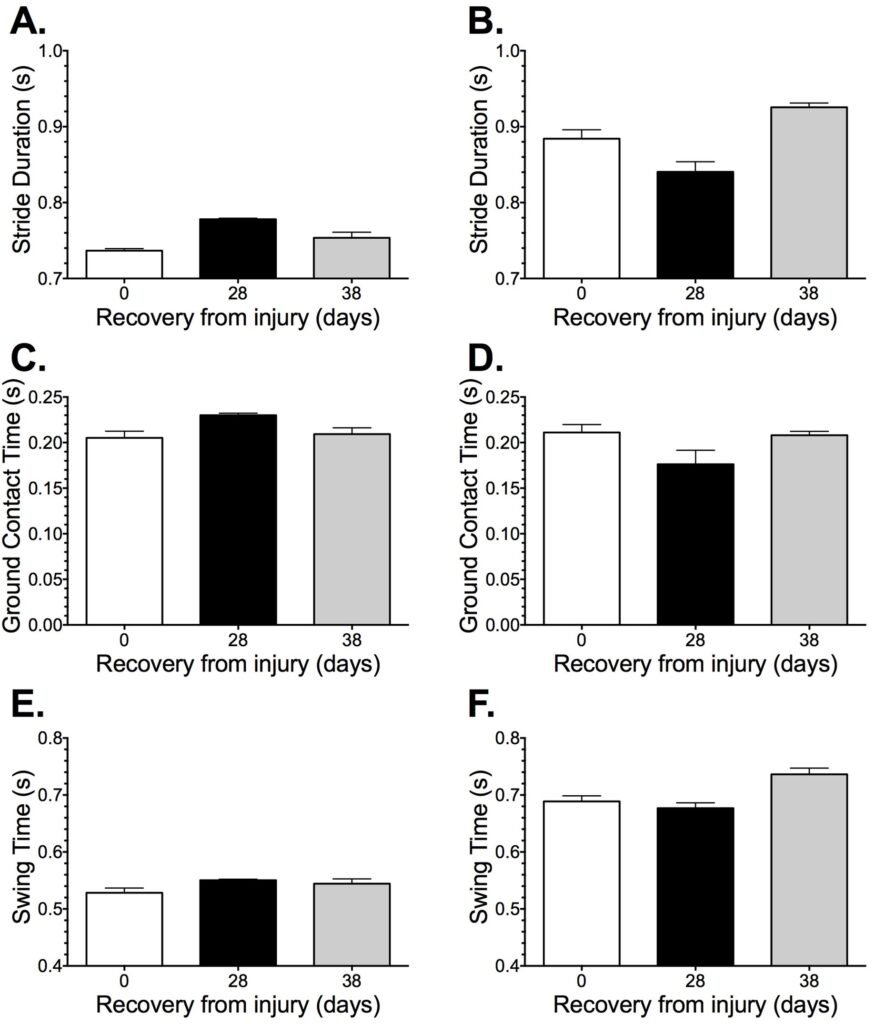
Where, A. Total stride duration (LTM), B. Total stride duration (ATM), C. Ground contact time (LTM),
D. Ground contact time (ATM), E. Swing time (LTM), F. Swing time (ATM).
Physiological Variables
Data analysis showed no changes over 5 min epochs for heart rate and V̇O2 within trials (Figure 4) but highlights considerable differences in work done between conditions (LTM and ATM). Overall trial analysis identifies a reduction in average heart rate for both conditions (LTM and ATM) following the period of ATM training in both the 28 d and 38 d trials when compared to the pre-injury trial (102±1, 104±1, and 107±1 for LTM; 127±1, 121±1, and 121±2 for ATM), respectively. While there was an initial decrease in V̇O2 values from pre-injury (0 d) to 28 d, the 38 d trial suggests a return to pre-injury values (38.6±1.4, 31.2±0.8, and 36.1±1.0 for LTM; 55.0±1.0, 40.9±0.3, and 48.7±1.6 ml·min-1·kg-1 for ATM), respectively.
Figure 4. Mean physiological data for each 5 min epoch within each trial (reference time point in days
since recovery from injury) and separated for condition (LTM and ATM) for: A. Heart rate LTM;
B. Heart rate ATM; C. V̇O2 LTM; and D. V̇O2 ATM.
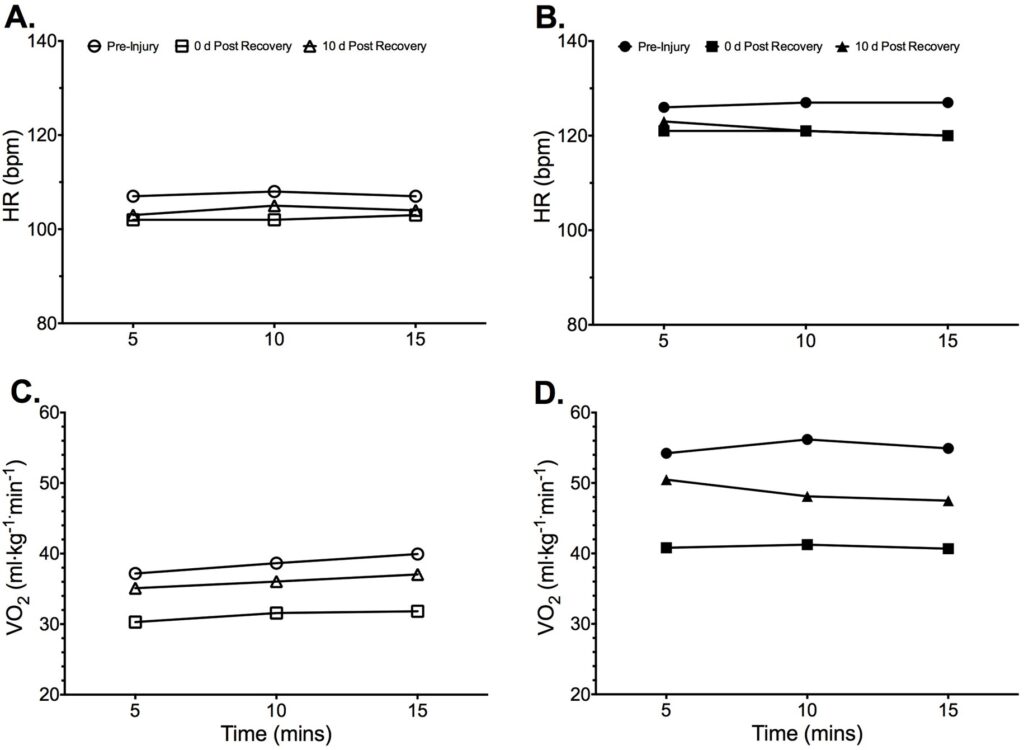
As there were no differences between 5 min time periods and conditions it was decided to combine physiological data within conditions (LTM+ATM). This analysis (Table 1) highlights the overall differences between trials in relation to pre-injury testing (0 d) and the two trials post-rehabilitation (28 and 38 d). Key differences include an increased efficiency at 28 d post rehabilitation expressed through variables the variables: V̇O2,V̇E:V̇O2, heart rate, oxygen pulse and V̇O2 per stride (Table 1). While, after a 38 d resumption of normal land based training the aforementioned variables had returned to values similar to pre-injury (0 d) levels and thus showed significantly different to 28 d post rehabilitation (Table 1).
Table 1. Mean±SD for Overall (condition) Effect on Physiological Variables
|
0 d
|
28 d
|
38 d
|
O2 (ml×min-1×kg-1) |
46.85±9.11
|
36.07±5.33
|
42.37±7.02
|
E (L×min-1) |
78.77±18.39
|
78.59±23.06
|
74.64±15.53
|
E: O2 |
28.92±1.42
|
36.45±5.57
|
30.03±1.34
|
Rf (b×min-1) |
39±1
|
40±4
|
35±3
|
Tidal Volume (L) |
2.03±0.48
|
1.91±0.38
|
2.09±0.26
|
HR (bpm) |
117±11
|
112±10
|
113±10
|
O2 Pulse (ml×bpm-1) |
22.92±2.44
|
18.91±1.12
|
21.80±1.79
|
O2 per stride (ml) |
0.62±0.18
|
0.48±0.09
|
0.58±0.16
|
No. Strides per Breadth |
1.93±0.22
|
1.86±0.27
|
2.08±0.41
|
DISCUSSION
The aim of this brief report was to establish the efficacy of waist depth ATM running throughout a period (28 days) of injury to the lower leg, where normal land-based running was not possible. As such the emphasis was to assess and compare post-injury LTM running spatio-temporal parameters and the subsequent physiological responses with pre-injury measures. The main findings were: (a) Spatio-temporal parameters (stride duration tc and tsw) increased during LTM and decreased for ATM following the rehabilitation period; (b) Improvements in measures pertaining to physiological efficiency following the rehabilitation phase of ATM training; and (c) Spatio-temporal and physiological changes showed signs of returning to pre-injury status following 10-d of normal land-based training.
Under normal circumstances the injury experienced would mean that the participant would have had to stop all running activity. However, the water treadmill enabled training at a reduced level and as such could be classified as a taper rather than an injury caused cessation (Figure 1). In agreement with running specific tapering strategies used by Olympic athletes to enhance performanc.33 The participants sustained 60-70% of their normal dry land pre-injury training volume (Figure 1), but excluding the normal. High intensity interval training including in such tapering protocols.33 It is possible that cessation of such work could affect maximal physiological capacity, spatiotemporal metrics and the associated measures of performance. However, due to potential for re-injury such training was not recommended or tested.
The data collected in this case study supports the increased metabolic cost of running during ATM at the same speed (Figure 4) and is likely a result of increased hydrodynamic resistance,26 identified as an “added-mass”.32
Analysis of the time course data prior to and following injury provides contrasting results with regards to technical changes highlighted by spatio-temporal metrics (Figure 3). The ATM condition suggests adaptation to more efficient water immersed running technique following prolonged exposure to ATM training without land-based training. This would occur in order to overcome hydrodynamic resistance exposure during ATM26 and is reflected by physiological values associated with metabolic efficiency (Table 1). The change in running technique during ATM condition following injury also coincided with changes to LTM running. This included an, increased stride length primarily due to increased ground contact time, and coincides with reduced metabolic cost of LTM running following a period of ATM training. It is proposed that the increased resistance to limb extension occurring during ATM afforded the participant greater force production capability of the limb extensors but requires further research.34,35
Importantly, the return to land training (LTM) resulted any changes as a result of the ATM rehabilitation period quickly reverted to pre-injury values. This suggests that athletes requiring several weeks of rehabilitation from injury could utilize water treadmill training as a means of specific supplementary training. The benefits of which would include, reduced re-injury risk with no negative impact on running mechanics or submaximal physiological capability.
CONCLUSION
The results of this case study suggest that 28 days of water treadmill running immersed to the anterior superior iliac, improved physiological response to sub-maximal exercise. As such, water treadmill running is a worthwhile alternative to land-based running during periods of injury where impact forces inhibit rehabilitation.
PRACTICAL APPLICATION
This work supports the use of ATM as a rehabilitatory training method while maintaining cardiovascular fitness during periods of injury where normal land-based running is not possible. Additional supplementary use in non-injured athletes may also benefit improvements in spatio-temporal parameters and running efficiency without increased injury risk typically associated with increased training load. This latter aspect requires further investigation.
CONFLICTS OF INTEREST
The authors declare that they have no conflicts of interest.