Bone fragility fractures due to deteriorated tissue quality are a major healthcare concern in the healthcare of elderly who are at particularly high risk of bone fractures. Thus, identifying and treating patients at risk is critical in sustaining a healthy life style for the elderly. Currently, bone mass or Bone Mineral Density (BMD) is commonly employed by physicians as a measure to predict the risk of such fractures. However, BMD is often a poor predictor of overall bone quality,1 showing that patients with healthy BMD levels may still be susceptible to fragility fractures.2,3 Hence, reliable prediction of bone fragility fractures requires understanding the underlying origins of bone fragility fracture beyond the qualitative estimation provided by BMD measurements.
Many biological materials exhibit a recurring motif of hierarchical structures, which is optimized by nature to adapt to surrounding environments (e.g. mechanical loading). In general, the key attribute of the hierarchically structured materials is that their bulk behavior is not simply a function of the mechanical properties of their primary constituents, but also of the shape and arrangement of these constituents at different length scales.4 These constituents are integrated to form basic building blocks at nanoscopic levels, which are then further arranged to form building blocks at higher length scales. Similarly, bone as the primary load bearing tissue in the human body also exhibits a hierarchical structure.
Among the different hierarchies of human bones, lamella is the fundamental building block, which is a sheet like structure comprising parallelly arranged mineralized collagen fibrils surrounded by an extra-fibrillar matrix (EFM) (Figure 1). Advanced microscopy techniques demonstrate that mineralized collagen fibrils consist of mineral crystals orderly arranged within collagen fibrils.5,6 Several structural models of mineralized collagen fibrils are proposed based on the experimental observations and exhibit the efficacy to capture the key features of the arrangement and the mechanistic behavior of its moieties.7,8,9 However, the less explored and understood is the EFM in bone lamella.
Figure 1: Schematic of bone ultrastructure.
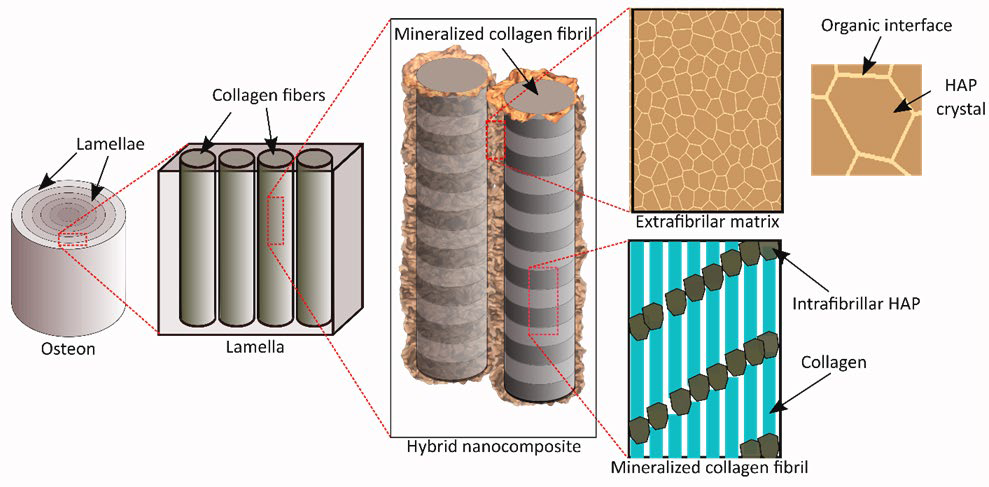
Differing opinions have been raised by researchers on the structure and arrangement of mineral crystals in and around the collagen phase. Nonetheless, there exists a general consensus that “plate-like” mineral crystals exist both in the gap regions of collagen fibrils to form mineralized collagen fibrils and also outside the fibrils as part of the EFM. In fact, recent Transmission electron microscopy (TEM) results reinforce this conjecture, showing that lower density regions were embedded in a higher density region at nanoscopic levels,5 thereby indicating that the softer collagen fibrils are surrounded by harder mineral crystals. Thus, it could be assumed that mineral crystals are a major component of the EFM in bone.
Previous evidence shows that bone fragility may originate at very small length scales,10,11 ranging from nano to submicron length scales. The effect of ultrastructural alterations is propagated to higher length scales and eventually results in bulk fragility fractures. For instance, water has been known to be a plasticizer of bone. In the absence of water, bone would fail in a brittle fashion.8 However, the underlying mechanism is still unclear. Nonetheless, recent evidence exhibits that the plasticity and toughness of bone is dependent only on a small fraction of water that occupies small ultrastructural ‘pores’ in bone,12 while water in the larger anatomic pores (e.g. Haversian canals, lacunae, and canaliculi) shows very limited contributions to the bulk mechanical properties of bone. The size of the ultrastructural space is identified as less than 4 angstroms, thus making other molecules smaller than water not penetrable into the space. This is an indication that the effect of water on bone toughness is manifest only at the molecular level rather than the structural voids in the tissue.
Recent studies have made promising strides towards deciphering the role played by water and the extra-fibrillar mineral matrix in sustaining the toughness of bone. New evidence indicates that removal of proteoglycans from the EFM in bone may lead to significant decreases in bone toughness and this effect is coupled with the hydration status of the matrix.13 One possible scenario is that the mineral crystals in the EFM are bounded through a network of highly hydrophilic non-collagenous proteins (i.e. proteoglycans), which are capable of drawing in and trapping water molecules in the matrix. The hydrated organic interface makes the mineral crystals slide between each other much easier, thus imparting the plasticity and toughness to the tissue.8 Computational simulations of the extra-fibrillar matrix model with the organic interface also support the speculation, showing a close match between the predicted and experimentally (i.e. synchrotron X-ray diffraction) observed behavior of the mineral phase in bone (Liqiang L, et al. unpublished data, 2016). The computational simulation results also indicate that bone is brittle under “dry” condition, but is appreciably ductile under “wet” condition, which are in good agreement with the experimental observations.
Some important inferences that can be drawn from the aforementioned results are: First, the extra-fibrillar matrix plays a pivotal role in the mechanical behavior of bone tissues. Second, hydration is necessary for the extra-fibrillar matrix to sustain large strains, thereby imparting toughness to bone. Third, non-collagenous proteins such as proteoglycans are potentially a pivotal ultrastructural component that dictate the toughness of bone. In conclusion, the extra-fibrillar matrix in bone plays a significant role in the toughness of bone.
CONFLICTS OF INTEREST: None.