INTRODUCTION
Multiple sclerosis (MS) is an immune-mediated disease of the central nervous system. MS is characterized by demyelination, inflammation, axonal loss, and gliosis. Demyelination leads to decreased nerve conduction producing a cascade of symptoms such as loss of motor function and abnormalities in sensation. Experimental autoimmune encephalomyelitis (EAE) is one of the most widely used animal models of MS that can be induced in the central nervous system through injections of myelin basic protein (MBP).1,2,3,4 Administration of MBP causes the production of destructive antibodies that target the myelin sheath surrounding the spinal cord. This immune response is responsible for the development of symptoms like those seen in human patients with MS.
The approved drug therapies for MS are highly toxic and can cause adverse side effects such as depression, heart failure, liver problems, and increased risk of infection.1 These therapies include immunosuppressants and are mainstays of conventional MS management. It is therefore important to explore alternative treatment options to those currently available. Oxygen therapy, including hyperbaric oxygen (HBO) and normobaric oxygen (NBO), has been associated with therapeutic benefits in various disease states. NBO is the medical use of oxygen at sea-level pressure, whereas HBO is the medical use of oxygen higher than atmospheric pressure.5,6 The mechanism(s) by which both treatments work, while not completely understood, is fundamentally through increased oxygen uptake by damaged tissues. Previous preclinical research has shown HBO to be effective in decreasing symptoms associated with animal models of neuropathic pain, arthritis, and inflammatory pain, among others7,8,9,10,11 while other preclinical research has shown NBO to be useful in decreasing symptoms associated with carbon monoxide poisoning and cerebral ischemia.12,13,14,15,16 Clinical studies have also demonstrated a degree of therapeutic benefit with HBO treatment in selected subgroups of patients with MS.17
Considering these findings, it may be possible that NBO or HBO could alleviate the symptoms of EAE through regularpressure or high-pressure oxygen. If shown to be successful, NBO or HBO could provide relatively safer alternatives to current pharmacotherapies that are administered to human patients with MS and might lead to further understanding of the mechanisms of this disease. Therefore, the purpose of the present study was to determine if NBO or HBO could attenuate the motor and sensory alterations that develop following induction of EAE.
MATERIALS AND METHODS
Subjects
In the current study, forty-two male Harlan Lewis rats weighing approximately 225-250 g were used. Animals were singly housed in the University of Texas at Arlington vivarium on a 12-hour light/ dark cycle with food and water available ad libitum. Approval of the protocol was obtained from the University of Texas at Arlington Institutional Animal Care and Use Committee (A12.011, July 30, 2015), and the experiment was conducted in accordance with “The Ethical Guidelines for Investigations of Experimental Pain in Conscious Animals”.18
Materials
Induction of experimental autoimmune encephalomyelitis: EAE was induced in the central nervous system via injections of MBP emulsified in complete Freund’s adjuvant (CFA). MBP (Sigma-Aldrich) was prepared in solution using 100 μg protein in an equal volume of CFA (4 mg/ml Mycobacterium tuberculosis) dissolved in 100 μL of phosphate-buffered saline (0.1 M phosphate-buffered saline, pH 7.3; 50% g/v). Under isoflurane anesthesia (3% induction/2% maintenance) animals received an intracutaneous injection of the emulsion in the spinal region between thoracic 12 (T12) to lumbar 1 (L1) on each side lateral to the backbone. Each rat received a total of 0.2 mL of emulsion for a total antigen dose of 100 μg per rat.
Hyperbaric oxygen/normobaric oxygen treatment: HBO consisted of administering oxygen for 60-minutes daily at 2.0 atmospheres absolute, a pressure equivalent to 33 feet of seawater. Each treatment lasted for about 80-minutes, including approximately ten minutes on either side of the treatment for compression to “depth” and recompression to “surface”. The NBO group was placed inside the chamber for 60-minutes and received a continuous flow of 100% oxygen, but did not experience any changes in pressure. Oxygen flow rate was maintained via an oxygen flow meter at 5 L/min.
Mechanical paw withdrawal threshold testing: MPWT testing was conducted to assess for the presence of tactile allodynia. MPWT testing was done on the first day (day 1 baseline MPWT) and every other day after the start of treatment, beginning on day 8. MPWT was accomplished by stimulation with Von Frey monofilaments of varied force ranging from 3.85 to 251.34 mN. Subjects were placed atop a wire mesh floor inside Plexiglas chambers and allowed to habituate for 10-minutes. Stimuli were applied for one second to the plantar surface of each hind paw. Responses, or lack thereof, determined the pattern of application of the stimuli. Responses to the stimuli included licking, flicking, flinching that results in removal of the paw, and total withdrawal of the paw. Lack of a response resulted in the application of a higher force stimulus and presence of a response resulted in application of a lower force stimulus. MPWT was established using a formula that considers the pattern of responding, the force for the initial response, and force of the last stimulation.19 Higher MPWT scores reflected less sensitivity, whereas lower MPWT scores indicated greater sensitivity and discomfort. Three trials were conducted for each paw and the scores were averaged. The maximum, or rate at which there was no response or sensitivity, was 456.63 mN.
Motor impairment testing: The method of motor impairment testing was adapted from behavioral assays conducted by Pender et al.20 Animals were examined twice daily, once before and once after treatment. Tail weakness was assessed by holding the animal by the base of the tail and observing tail movement. It was graded as follows: 0=no weakness; 1=weakness of distal tail only, the distal tail failing to curl around the examiner’s finger; 2=weakness of the whole tail but with the proximal tail still being able to be erected vertically against gravity; 3=severe weakness with only a flicker of tail movement; 4=complete flaccid paralysis of the tail. Hindlimb weakness was graded thus: 0=no weakness; 1=slight dragging of the toes of the hindfoot; 2=severe dragging of the hindfoot but not of the rest of the hindlimb; 3=severe dragging of the whole hindlimb; 4=total paralysis of the hindlimb. If the hindlimb involvement was asymmetrical, the mean grade was used. Two raters, “blinded” to treatment conditions, were used to acquire behavioral assay scores for tail and hindlimb weakness. One composite score measuring both tail and hindlimb weakness was taken from each animal twice daily from each observer, and these ratings were then averaged.
Experimental Protocol
Animals were randomly assigned to one of four conditions: MBP/ HBO, MBP/NBO, MBP/no treatment, and vehicle. Injections of MBP were administered on day 1, and animals were allowed a week to recover. Baseline MPWT scores were taken on day 1. Following recovery, on day 8, HBO/NBO treatment was started. MPWT testing was conducted on day 8 and every alternate day after (day 10, day 12, day 14, day 16, and day 18). Behavioral assay scores were acquired each day, before and after treatment. HBO/NBO treatment continued until day 19 when the protocol ended and animals were sacrificed.
Data Analysis
The current experiment employed a between-subjects design. For the MPWT data, a mixed-design repeated measures analysis of variance (ANOVA) was conducted with time as the within-subjects factor and condition as the between-subjects factor. For the motor impairment data, nonparametric Kruskal-Wallis tests were conducted to evaluate differences among the four experimental conditions. Follow-up Mann-Whitney U tests were done to evaluate pairwise differences among the groups when values were significant (p<0.05). The data from each experiment was analyzed in SPSS v.22.0.0.0, and graphs were created using SigmaPlot v.12.3.
RESULTS
Results from MPWT data indicated a significant main effect of time on the average MPWT scores, F(13,494)=4.95, p<0.001, with sensory thresholds increasing over time, indicating a lack of tactile allodynia. However, there was a non-significant main effect of condition on the average MPWT scores, F(3,38)=0.85, p=0.48, nor a significant interaction of time and condition on average MPWT scores, F(3,494)=1.16, p=0.24 (Figure 1).
Figure 1. Mechanical Paw Withdrawal Threshold (MPWT) Test Results
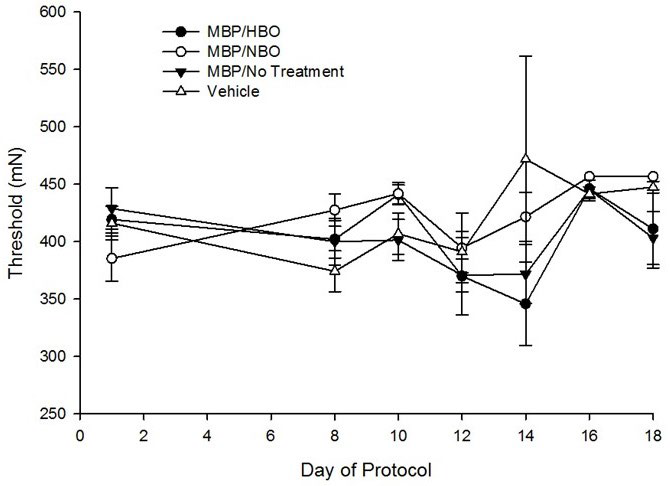
For the motor impairment scores (Figure 2), data were first analyzed by looking at scores from animals injected with MBP against animals injected with vehicle. A Kruskal-Wallis test showed that there was a statistically significant difference in motor impairment scores between the animals injected with MBP and animals injected with vehicle on day 14, χ2 (1)=4.44, p=0.035, with a mean rank motor impairment score of 24.12 for the MBP group and 17.65 for the vehicle group. The group with the lowest mean rank corresponds to the group with the greatest number of lower scores, while the group with a higher mean rank has higher scores. On day 15, there was a statistically significant difference between MBP and vehicle groups, χ2 (1)=8.52, p=0.004, with a mean rank motor impairment score of 24.9 for the MBP group and 16.5 for the vehicle group. On day 16, there was a statistically significant difference between MBP and vehicle groups, χ2 (1)=3.96, I=0.047, with a mean rank motor impairment score of 23.9 for the MBP group and 17.97 for the vehicle group. On day 17, there was a statistically significant difference between MBP and vehicle groups, χ2 (1)=8.51, p=0.004, with a mean rank motor impairment score of 24.9 for the MBP group and 16.5 for the vehicle group. Lastly, on day 18, there was a statistically significant difference between MBP and vehicle groups, χ2 (1)=5.24, p=0.022, with a mean rank motor impairment score of 24.26 for the MBP group and 17.44 for the vehicle group. The observed trends indicate that the groups injected with MBP had significantly higher motor impairment scores than the vehicle group, suggesting increased motor deficits.
Figure 2. Motor Impairment Score Test Results
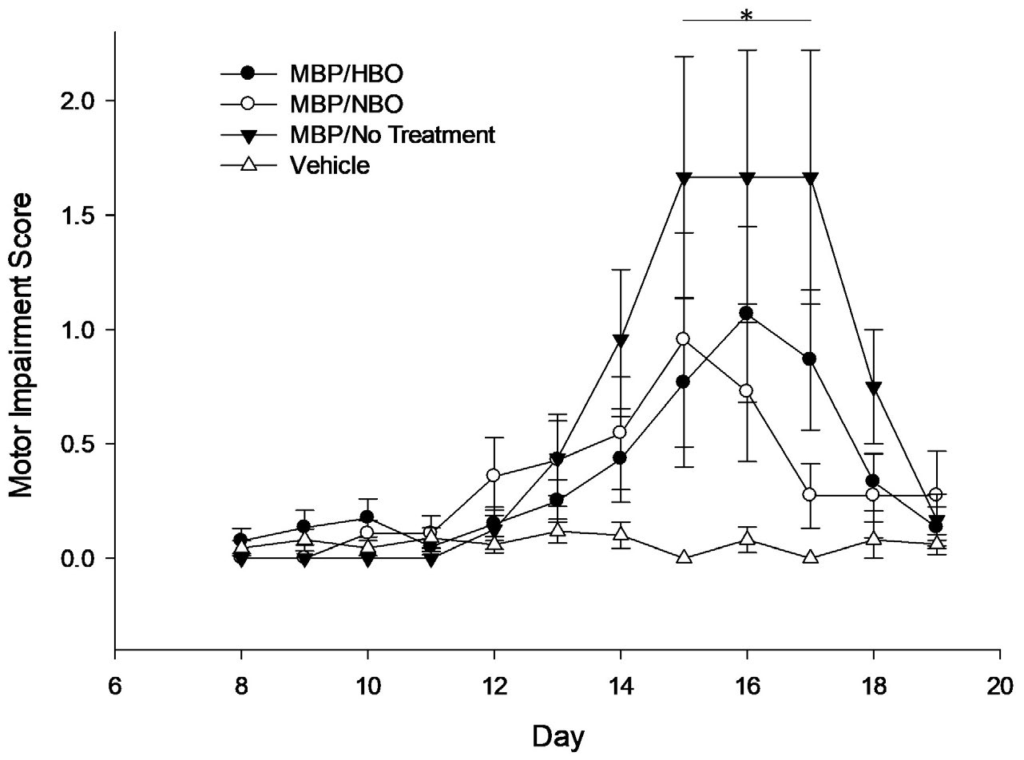
Next, motor impairment scores were examined by looking at the individual groups (MBP/HBO, MBP/NBO, MBP/no treatment, and vehicle). A Kruskal-Wallis test showed that on day 15, there was a statistically significant difference in motor impairment scores among groups, χ2 (3)=9.95, p=0.019, with a mean rank motor impairment score of 22.55 for MBP/HBO, 25.00 for MBP/NBO, 27.75 for MBP/no treatment, and 16.50 for the vehicle group. Similarly, on day 17, there was a statistically significant difference in motor impairment scores among groups, χ2 (3)=9.91, p=0.019, with a mean rank motor impairment score of 23.00 for MBP/HBO, 24.07 for MBP/NBO, 28.00 for MBP/no treatment, and 16.50 for the vehicle group.
DISCUSSION
We examined and described the efficacy of HBO or NBO treatment in decreasing the symptoms associated with EAE. MBP developed greater motor impairment compared to vehicle-injected animals. In addition, treatment with oxygen significantly decreased the magnitude and duration of the symptoms associated with MBP. It should also be noted that the MPWT sensory testing suggested that none of the groups developed tactile allodynia, as evidenced by the continuously high threshold values. The lack of robust allodynia may be cautionary for future researchers looking at this specific model of EAE, which used MBP as antigen and CFA as an adjuvant. Species differences in the animal model used may account for disparities in the amount of allodynia that develops.1 For example, Constantinescu et al1 asserted that EAE lesion pathology varies between animal strains. In particular, it has been shown that in the Lewis rat, EAE induced by MBP can produce severe inflammation of the CNS with little to no corresponding demyelination.21 Importantly, genetically identical animals may differ in susceptibility to induction of EAE.21 These factors explain the paucity of robust MPWT data in the current experiment.
The results from the motor impairment test indicated that the MBP/no treatment group experienced the most pronounced development of motor impairment, as expected. The group administered vehicle had the lowest motor impairment scores, which was also expected. On days 15 and 17 of the protocol, mean rank motor impairment scores indicated that the magnitude of motor deficits was worst in the vehicle group, followed by MBP/NBO, MBP/HBO, and lastly, lowest in the vehicle group. Interestingly, both treatment groups (MBP/HBO and MBP/NBO) not only had reduced motor impairment scores relative to the vehicle group, but also had a similar shorter duration of symptom presentation.
While the exact etiology of multiple sclerosis is not yet clear, there are mechanisms known to influence its development. For example, the integrity of the blood-brain barrier (BBB) is lost during multiple sclerosis. Penetration of leukocytes and other immune cells into the central nervous system (CNS) can modify the permeability of the BBB, and as more immune cells enter the CNS, the formation of reactive oxygen species (ROS) can occur.22 ROS are released by inflammatory cells and are mediators of demyelination and alterations to BBB permeability that is characteristic of MS.23 ROS exist in a variety of forms but are similar in that they cause damage through oxidative stress. According to Hsieh et al,24 ROS production is closely associated with the generation of nitric oxide (NO). Smith et al25 have noted that in patients with inflammatory MS, there are elevated levels of NO. Elevations in ROS have also been shown in models of EAE.26
The production of peroxides and free radicals can lead to cellular damage, and these processes can be ameliorated by antioxidants. Antioxidant therapy has been shown to reduce migration of monocytes across BBB endothelial cells and suppress symptoms in EAE.27,28 NBO has been shown to induce varying degrees of antioxidant enzyme production specific to NO, which would serve to lower levels of NO.12,13 NBO has also been shown to be effective in treating carbon monoxide poisoning and cerebral ischemia.14,29 HBO treatment, on the other hand, leads to increases in dissolved oxygen in the blood and upregulation of antioxidant enzymes.30
Although increased levels of NO are a purported mechanism in the development of MS, its effect is not always negative. According to Smith et al,25 NO production in MS also serves a beneficial immunomodulatory purpose. These authors have noted that this dual effect of NO in multiple sclerosis may explain why inhibiting NO (through NBO or HBO) has not led to more encouraging results in animal models of MS such as EAE.25 As an example, Ohgami et al8 showed that neural NO was necessary for the expression of the acute antinociceptive effect of HBO. This notion may also help explain why animals in the treatment conditions (MBP/HBO and MBP/NBO) in the current experiment did not experience greater attenuation of motor symptoms, as well as why the MPWT data were not more robust.
CONCLUSION
There has been limited work done recently using the EAE model. Chiou et al31 found that HBO attenuated EAE through the modulation of T-cell responses. However, the results of the present experiment lend credence to the notion that pressurization of oxygen, as used in HBO, may not be a necessary component in the reduction of symptoms in EAE. Overall, there remains a paucity of preclinical research comparing these two modes of oxygen delivery. However, in clinical research, the limited availability of HBO chambers and poor patient compliance may lead to difficulty implementing HBO in widespread use.32 Therefore, NBO may be an effective alternative treatment to HBO for the treatment of MS, among other disease states. The results from the current experiment serve to reinforce the need for further research using oxygen treatment. Future research directions involve the determination of the ideal parameters of oxygen delivery and assessing whether pressurization of oxygen is needed treating this specific animal model of EAE.
ACKNOWLEDGMENTS
We thank Michelle White for her help in the preparation of this manuscript and Dr. Christopher McNabb and Amber Harris for their assistance with behavioral testing as well as serving as observers for motor impairment tests. This work was funded by a University of Texas at Arlington Research Enhancement Project grant awarded to P.N.F. and J.R.W
CONFLICTS OF INTEREST
The authors declare that they have no conflicts of interest.