INTRODUCTION
Trauma is the leading cause of death in children over the age of one, and traumatic brain injury (TBI) is the leading cause of death and disability due to trauma, accounting for 70% of fatal injuries.1 In the United States, every year there are 3,000 deaths, 50,000 hospitalizations and 675,000 emergency room (ER) visits related to blunt head trauma.1 TBI is a major public health and socio-economic problem throughout the world, especially among children and young adults.2 Even in patients who do not have any recognizable initial clinical manifestations, it can still have devastating long-term consequences on neurocognitive function and psychosocial behavior, if untreated. The use of computed tomography (CT) has increased in recent years, thus enabling early identification of TBI. Accordingly, many recent studies have shown an association between lifetime risk of developing cancer and ionizing radiation from CT exams. This lifetime risk of cancer is higher in young children when compared to adults.3,4 Though CT has remained the mainstay imaging modality used in the initial evaluation of pediatric head injury, faster magnetic resonance imaging (MRI) techniques have proven to be more sensitive in identifying subtle findings of brain injury. Specifically, MRI has been used in differentiating subacute and chronic brain injury, and identifying the extent of encephalopathy, reactive gliosis, and hemorrhage related to the insult.5,6,7 In addition, MRI is increasingly being used in the acute setting for stable patients who have a discrepancy between clinical symptoms and CT findings.8
There are many potential long-term sequelae in pediatric patients who suffer TBI including, long-term physical or intellectual disability, memory problems, behavioral problems, and cognitive functioning issues. Children with moderate to severe TBI have poorer outcomes in terms of executive functioning (attention, problem-solving), intellectual functioning, and to a lesser extent visual perceptual functioning. In addition, patients with moderate to severe TBI have been noted to have substantially more issues with education and long-term outcomes. More research is needed to determine how to improve therapy or rehabilitation in patients with these consequential outcomes following head trauma. In the meantime, quantifying and recognizing imaging findings early and correlating those with clinical symptoms seems to be of clinical value and MR plays a crucial and growing role in that respect. In this literature review, we intend to present the current information about the use of MR in evaluating pediatric head trauma.
Epidemiology
It has been reported that between 100-300 per 100,000 children and young adults are hospitalized with a head injury each year,9,10,11 with many more outpatient and emergency department (ED) visits than admissions. In a 25-year longitudinal study from New Zealand, a birth cohort (n=1265) was found to have an overall prevalence of TBI of about 30%.12 Abusive head trauma (AHT) is an important cause of TBI to recognize. AHT is seen more commonly in patients younger than the age of two years, with incidence in that group being described as 17 per 100,000 person-years (95% CI, 13.3-20.7 per 100,000 person-years).13 Recognizing subtle features in the history or physical is imperative because infants with head injuries frequently have non-specific symptoms and often no history of trauma is provided. Injury could be a result of blunt force, shaking, or a combination of both. One retrospective chart review in the US found that AHT was not recognized in >30% of patients seen by a physician, and almost 13% of those were radiologically misinterpreted.14 Of those with no demonstrable injuries on initial evaluation, 28% were reinjured. A study from the UK concluded that 52% of the head injuries in kids <1 year of age was due to suspected assault.15 Although recognizing head trauma can be relatively difficult in the absence of a reliable patient history, suspicion of AHT should prompt one to consider using appropriate imaging studies to evaluate the brain for any structural damages. It is therefore vital for clinicians and imaging experts to be able to discern characteristic imaging features of AHT to inform the proper authorities about potential abuse.
Besides the obvious acute presentations of TBI in children, long-term consequences should be of clinical concern. Many studies have shown that severe pediatric TBI causes behavioral, cognitive, and academic deficits over the years.16,17,18 Therefore, it is logical to assume that if intracranial injuries are diagnosed and quantified earlier, timely intervention, rehabilitation and other appropriate measures can be instituted to improve outcomes in those affected.
Traumatic Brain Injury
TBI can be categorized using various parameters, including severity, physical mechanism of injury (penetrating or non-penetrating, blunt or blast), anatomic type of injury (focal vs. diffuse), pathophysiology (primary or secondary), neuroimaging findings, prognostic classification, and more. The severity of TBI has historically been classified based on the Glasgow Coma Scale (GCS) of the patient when initially evaluated. It is a cheap, reliable source for gauging a patient’s status using verbal responses, motor responses, and eye opening. GCS is a 15-point scale (table) that can be rapidly evaluated by a healthcare professional at the bedside of any patient and be used to guide acute management. Mild TBI is classified as a score of 13-15, moderate TBI is 9-12, and a score <8 on the GCS is considered severe TBI. However, the GCS does have its limitations; it is difficult to get a measure on very young patients (i.e. <2 years of age), as well as in patients who are intubated or have extracranial problems (such as electrolyte imbalance.). Also, due to the heterogeneous nature of some brain injuries, GCS does not provide details regarding the pathophysiology of neurologic deficits. In fact, nearly half of the patients with documented TBI on CT have GCS scores of 14 or 151. Therefore, neuroimaging plays a crucial role in determining prognosis and guiding the course of management. As imaging technology advances, there will be a greater opportunity to stratify patients into specific treatment cohorts that can improve long-term outcomes.
Pathophysiology of TBI can be broadly classified into primary and secondary causes. Primary TBI is the result of a direct blow to the head, rapid acceleration-deceleration injuries, penetration wounds, or blast injury – often; these types of injuries are readily identifiable at initial presentation. In contrast, the presentation of secondary TBI is complex because the symptoms are usually insidious in onset. Secondary TBI is due to one or a mix of several problems (electrolyte imbalance, hypotension, hypoxia and/or ischemia, or inflammatory response). On conventional imaging techniques including a non-enhanced CT (NECT), the imaging findings of secondary TBI can be can also often subtle or unapparent. In contrast, conventional and advanced MRI sequences could provide higher sensitivity for detecting minor changes of brain injury when compared to a CT.
Diagnosis
Estimating the extent of injury in TBI patients can be difficult without the appropriate imaging techniques. Techniques such as angiography or venography with CT or MR may also be used in some cases when evaluating the integrity of the vessels of the brain. The primary diagnostic tool for assessing children with acute moderate-severe head injury (GCS≤13) regardless of GCS score in Emergency Departments is NECT.19,20 Though serial CT scans may detect cerebral edema, MRI is more sensitive in detecting early cerebral edema.21,22 Imaging in patients with mild head injury (GCS 14-15) is more controversial due to the risk of exposure to ionizing radiation and the relative susceptibility of younger patients to potential side effects of radiation; therefore, it is prudent to know which patients to send for a CT scan. There have been recommendations provided for head CT in children with very low risk of clinically-important TBIs, based on a prospective study by the Pediatric Emergency Care Applied Research Network (PECARN).1 There are no available established guidelines for the use of MRI post-TBI, however, this is a subject of active clinical investigation.
For subacute and chronic TBI, MRI is recognized as the most appropriate initial examination.20 The latter may especially be important in cases of AHT, since MRI allows for better dating of intracranial abnormalities, as well as stratify the degree of injury, and thus, may be relevant in determining the temporal course of brain injury (Figure 1). The features most distinguishing to recognize for inflicted TBI include, evidence of preexisting brain injury and retinal hemorrhages on MRI. It has been validated to be more sensitive than CT in detecting various phases of intracranial bleeding, and abnormalities of the posterior fossa and brain stem – especially with more advanced techniques including susceptibility-weighted imaging (SWI), and diffusion-tensor imaging (DTI).23,24,25,26 Moreover, the lack of beam-hardening artifacts in MRI permits better assessment of the brain stem, posterior fossa, and cortical surface.27 Newer advanced fast imaging techniques like parallel imaging include faster image acquisition, which can be used, for instance, to shorten breath-hold times resulting in fewer motion-corrupted examinations.28 The two parallel imaging methods most commonly used on clinical scanners today, sensitivity encoding (SENSE) and generalized auto calibrating partially parallel acquisitions (GRAPPA), both of these have significantly reduced scan times and therefore have added value especially in pediatric imaging where motion is critical factor in generated good quality images. Additional advanced MRI sequences like simultaneous multi slice imaging are available but beyond the scope of current discussion.
Figure 1. Axial T1(a) and T2 (b) Weighted Images Demonstrate Bi-Frontal Chronic Subdural Fluid Collections (Low T1, High T2) with Foci of Low T2 in the Left Parietal Collection Supportive of Foci of Acute on Chronic Subdural in a Patient with History of Non-Accidental Trauma
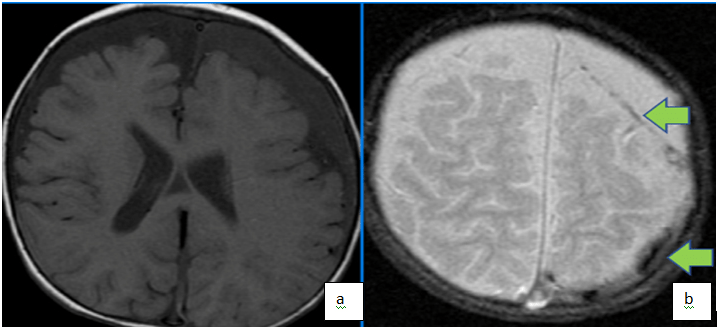
Skull Fractures
Direct impact to the head may cause fracture of the calvarial bones. Various patterns of fractures can be seen, including linear (or comminuted), depressed, and basilar skull fractures, in descending order of frequency. Parietal bone is the most commonly fractured bone in the calvarium, followed by the occipital and frontal bones. Most small linear skull fractures don’t have underlying parenchymal injury, and usually heal well without major complications, while patients with depressed skull fractures are more likely to have TBI.29 Epidural are associated with parietal bone fracture and consequent tearing of the middle meningeal artery. Sequelae from basilar skull fractures include dural tear with associated cerebrospinal fluid (CSF) leak, impingement of a cranial nerve, and cranial nerve deficit. Growing skull fractures are the result of large linear fractures that appear “growing” due to underlying tear of the dura mater and herniation of brain. As in most cases of TBI, NECT is the primary imaging modality of choice. Sensitivity of CT is increased with the use of three-dimensional (3D) reconstructed CT images, especially in cases of linear fracture.30 Ultrashort echo time projection reconstruction MRI has been described to be as sensitive as CT to identify skull fractures31 (Figure 2). MRI is also used in the analysis of growing skull fractures, which can better delineate the dural tear and bulging brain tissue.32
Figure 2. Axial NC, CT with Bone Windows (a) Demonstrates a Right Parietal Bone Fracture with Overlying Soft Tissue Swelling in a 5month Old That Fell from Mothers Lap. Axial Gradient Echo Image (b) Also Shows the Right Parietal Fracture. Note the Underlying Intracranial, Extra Axial Hemorrhage on the Axial Flair Image (c)
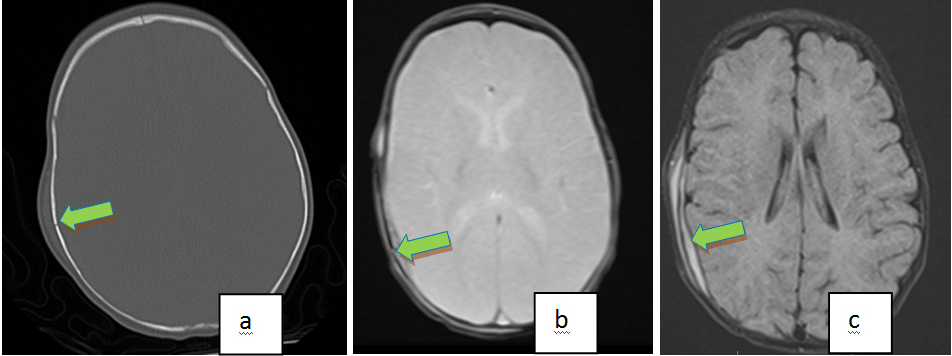
While MR is more sensitive in detection of most types of head trauma, CT with 3D reconstruction appears to be best modality for detecting skull fractures. MRI can be used as a screening tool in minor head injury (in conjunction with clinical exam and history) to identify parenchymal abnormalities and suggest the need for a CT scan. MRI can also be useful when the fracture itself is not well demonstrated on the MRI and in the presence of certain patterns of parenchymal injuries that occur with skull fractures. This could allow for the judicious use of radiation in the pediatric population while increasing the positive predictive value of the CT in detecting skull fractures for specific populations.
Intracranial Hemorrhage
Intracranial hemorrhage (ICH) is one of the common intracranial abnormalities following traumatic head injury; rapid imaging is required for early diagnosis, optimization of treatment, thus minimizing the potential for detrimental sequelae. ICH can be subdivided into extra-axial (epidural, subdural, or subarachnoid hemorrhages) and intra-axial (intraparenchymal and contusions), according to the anatomic location of the bleed relative to the brain. ICH can be assessed with ultrasound (US), CT, or MRI. Ultrasound is useful before the acoustic windows close (typically around 12 and 2-3 months for the anterior and posterior fontanelles, respectively). CT is quite sensitive and specific for elucidating acute hemorrhage and is enough to guide initial therapy. However, MRI has superior sensitivity for subtle hemorrhage and can provide the age of the hemorrhage. MR is particularly more sensitive in subacute injuries in comparison to CT. Each stage of ICH has a different appearance on MRI, as described on Table 1.33 Because of these differences in signals, MRI is beneficial in staging the examination.
Table 1. Age of the Hematoma as Seen on MRI |
Stage |
Duration |
Content |
T1 signal |
T2 signal |
Hyper acute |
Less than 24 hrs |
Intracellular oxyhemoglobin |
ISO |
Iso to hyper |
Acute |
1 to 3 days |
Intracellular deoxyhemoglobin |
ISO to low |
Low |
Early subacute |
3 to 7 days |
Intracellular methemoglobin |
Hyper |
Low to Iso |
Late subacute |
1 week to 4 weeks |
Extracellular methemoglobin |
Hyper |
Hyper |
Chronic |
After 4 weeks |
Hemosiderin |
Low |
Low |
Of the many types of MRI techniques, susceptibility-weighted imaging (SWI) has been shown to be more sensitive than gradient recalled echo (GRE) sequence in the detection of ICH due to the ability to accentuate paramagnetic properties of hemoglobin blood products, therefore more clear depiction of micro hemorrhages.21 SWI sequence has a longer acquisition time when compared to GRE and hence may be limited in use in the acute setting, especially in a moving child.
Epidural
Epidural hematoma (EDH) are characterized by an accumulation of blood between the skull and the periosteal layer of the dura mater (Figure 3). It can be caused by laceration of one of the meningeal arteries (most commonly the middle meningeal artery) but in children it is more frequently due to tearing of the dural venous sinuses.34 The onset of clinical manifestations may be delayed (“lucid” period) so rapid detection can be life-saving in these patients. Though venous EDH develop more slowly, arterial EDH expand more rapidly and can cause brain herniation (due to rising intracranial pressure) or ischemic injury if not diagnosed in a timely manner. Since the dura tightly attaches to the skull, EDH do not cross the cranial sutures. EDH most commonly appears on imaging as a biconvex lens-shaped extra-axial collection. If large enough, the lesion can cause a mass-effect with subsequent brain herniation. Small epidural can be missed on CT imaging due to volume averaging along the skull/CSF interface.
Figure 3. Axial Gradient Recall Echo (GRE) (a) and Coronal T2 Weighted (b) Images Showing Subacute Right Parietal Epidural Axial T1 Image (c) Shows High T1 Signal from Subacute Blood
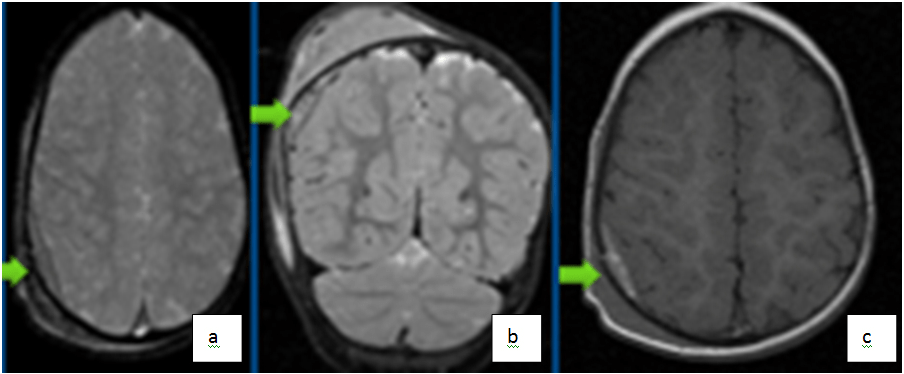
Subdural
Subdural hematoma (SDH) is a sequela of hemorrhage in the layer between the dura and arachnoid membranes, most commonly source is the bridging veins that run in from the subarachnoid space to the dural sinuses. Generally, it is found on the “contra-coup” side, in contrast to EDH, which is more commonly seen on the “coup” side. SDH may be seen in up to 80-90% of deliveries at term, especially when instrumentation such as forceps or vacuum extraction are used.35,36 The most common cause of SDH in children <2 years of age is average handle time (AHT).37,38 SDH carries a poor prognosis in many cases, three-quarters of these patients die or have significant disability due to direct compression or rise in intracranial pressure with subsequent herniation.39 SDH differs in appearance from EDH in that the former can cross cranial sutures (but is still limited by the internal dural attachments), therefore it typically is seen as a concave, crescent-shaped lesion on imaging. Involvement of the posterior fossa, or at multiple locations is a red-flag for AHT and the proper actions need to be taken to assure child welfare. MRI is the preferred technique in subacute SDH, since the lesions appear hyperintense on T1 and T2-weighted images (Figure 4). Fluid attenuated inversion recovery (FLAIR) is the best technique for demonstrating small SDHs, especially in cases missed by CT.40 Chronic SDHs are encapsulated and are characterized by their crescent-shape and low attenuation, ossification or vascularity of the lesion may be noted. A mixed pattern of attenuation could be due to new bleeding in an old site and could be suggestive of AHT. MRI is superior to CT in identifying the age of various blood products and thus, may be relevant in determining the temporal course of brain injury.
Figure 4. Axial T1 (a) and T2 (b) Weighted Demonstrates Bifrontal Chronic Subdural (Low to Intermediate Signal on Both T1 and High T2), Whereas High T1 Signal Posterior (Bilateral Parietal) Subdural Collections Represent Acute Subdural Hemorrhages. Note the Blooming from Chronic Blood Products on the T2* Star Sequence (c)
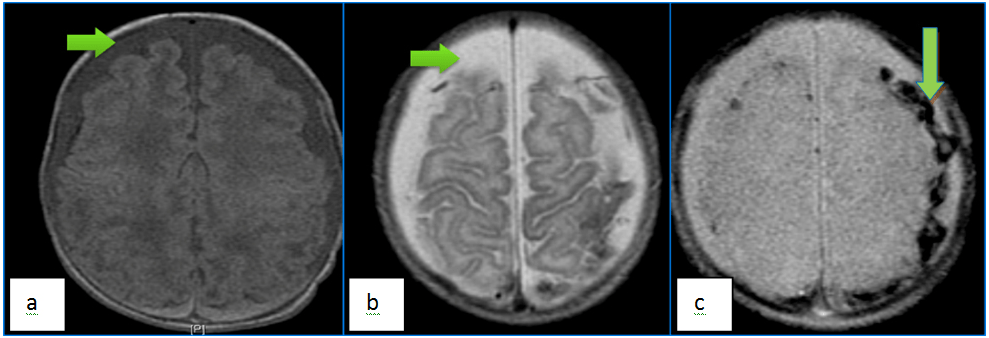
Subarachnoid Hemorrhage
Subarachnoid hemorrhage (SAH) is the accumulation of blood within the subarachnoid space, just superficial to the pia mater. It can be caused by injury to leptomeningeal vessels, or intraventricular bleeding with reflux into the subarachnoid space. A common site for SAH after head trauma is within the interpeduncular cistern or the lateral fissure.34 Non-enhanced CT is the modality of choice for acute SAH due to its sensitivity in detecting blood. Cerebrospinal fluid (CSF) dilution of the hemorrhage causes difficulty in recognizing SAH. MRI study using the FLAIR technique has been shown to have a sensitivity at least equal to CT for the detection of acute SAH27,41 (Figure 5).
Figure 5. Axial NECT (a) Shows An Acute Subarachnoid Hemorrhage in the Right Superior Temporal Gyrus. Axial Susceptibility Weighted Images (SWI) (b) Also Demonstrate Right Superior Temporal Gyrus Subarachnoid Hemorrhage. In Addition, a Countercoup Parenchymal Contusion is Noted in the Left Temporal Lobe (c)
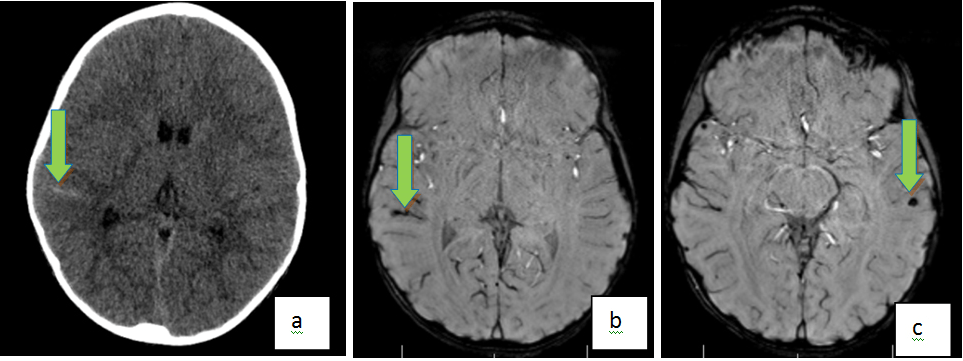
Intraventricular Hemorrhage
Intraventricular hemorrhage (IVH) can have several causes, including tearing of the sub ependymal veins, or reflux from a SAH. Cerebral bleeding may also leak into the ventricular system causing IVH. FLAIR and DWI are highly sensitive MRI techniques in the detection of subacute IVH. Hemosiderin deposition in subacute to chronic hemorrhages may be seen as T2 hypo-intense lining along the ventricular system.35
Parenchymal Injury
Parenchymal injuries include cerebral edema, cerebral contusions, diffuse axonal injury (DAI), and anoxic brain injury. Inherent high tissue resolution on MRI offers a more robust visualization of small parenchymal abnormalities. MRI has been shown to be more sensitive than CT in visualizing these types of brain injuries.6,42,43 MRI sequences with the greatest yield are gradient echo, fluid-attenuated inversion recovery (FLAIR) and DWI which should be included in all intracranial hemorrhage patients who undergo MRI.44
Cerebral Edema & Contusion
Cerebral contusions are a type of intracerebral hemorrhage that occur when direct impact to the skull causes trauma to the underlying brain and laceration of the intra-parenchymal vessels (Figure 6). It is most commonly due to non-inflicted trauma.45 Large lesions are easily discernable with US in neonates, and with CT in older children. However, MR is more sensitive in detecting small contusions especially using SWI sequence. Contusions are usually found in the deep layers of the cerebrum and delayed findings are not uncommon, thus it is prudent to suspect this in cases when neurologic condition worsens. Large parenchyma in the acute phase are better evaluated on non-enhanced CT. In the subacute stage, parenchymal can have a ring enhancing appearance. In the absence of a well-documented history of trauma in children, this appearance may be indistinguishable from other etiologies of intracranial ring enhancing lesions.
Figure 6. Right Temporal Hemorrhagic Contusions in a 16 Year Old Following Motor Vehicle Injury. Axial NC CT Image (A) Shows a Subtle Hyperintense Focus in the Right Temporal Lobe. Axial FLAIR (b) and GRE (c) MR Images Better Demonstrate the Blooming Right Temporal Hemorrhagic Contusion
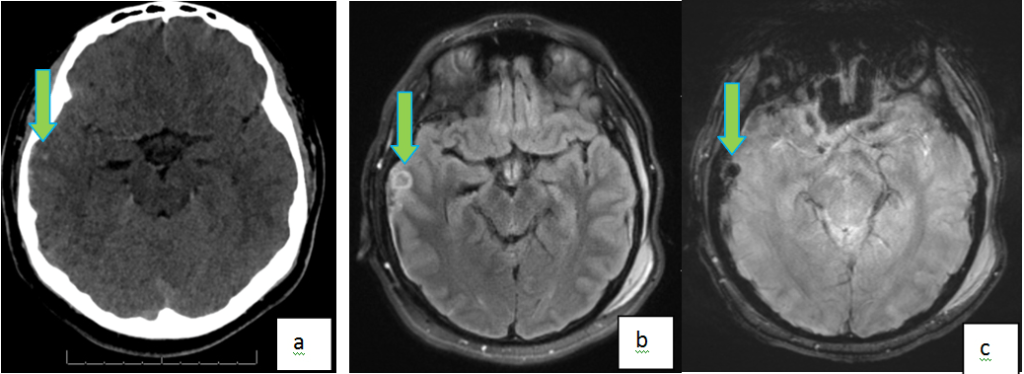
Cerebral edema can occur due to a combination of both vasogenic and cytotoxic mechanisms. It is a feared complication following TBI since it is the most significant predictor of outcome.21 DWI has been extensively used to evaluate cerebral edema and can provide valuable clues to the underlying mechanism46,47 (Figure 7). Early detection and treatment is aimed at lowering ICP to decrease mass effect and potential ischemia and/or herniation.
Figure 7. Coronal T2 FLAIR (a) and Axial T2 (b) Images Demonstrate Diffuse High FLAIR And T2 Signal in Bilateral Cortices from Edema. Axial DWI Image (c) Demonstrates Cortical High DWI Signal Indicative of Edematous and Ischemic Changes as Well in a Patient with Post Traumatic Hypoxic Injury
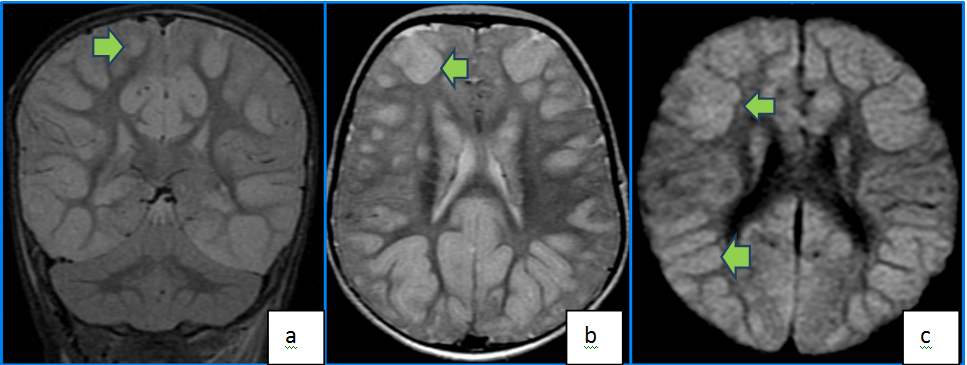
Diffuse Axonal Injury
Diffuse axonal injury (DAI) is one of the most common intra-axial injuries related to trauma; it is often a shear injury of the neuronal axons in white matter caused by acceleration-deceleration or angular forces (Figure 8). There is much research done on the clinical applications of MR for evaluating DAI cases, and it is the recommended modality for evaluating DAI.24,48,49 Sequences used include T1-W, T2-W, T2-gradient echo, FLAIR, DWI, and SWI. DWI and SWI have been shown to be helpful in delineating cases of DAI, which would not normally be seen in CT or conventional MRI techniques.50 On MRI, DAI is characterized by multiple, hyperintense T2/FLAIR lesions usually between 1 to 15 mm in diameter, caused by microbleeds and local edema. In the acute stage, both hemorrhagic and non-hemorrhagic contusions can show restricted diffusion on DWI sequence. The most common regions affected include the corpus callosum, internal capsule, and fornix. DAI should be suspected in patients in whom symptoms are more severe than what is seen on CT. Though not a neurosurgical emergency, presence of DAI are associated with worse clinical outcomes in patients with TBI51 and thus may warrant early detection & quantification. DAI is divided into three grades based on anatomic distribution in the brain. Grade I affect the gray-white interface. Grade II affects the corpus callosum in addition to the Grade I locations. In addition to the areas affected by Grade I and Grade II, Grade III lesions involve the brainstem. These grades are directly correlated to the clinical outcome. Unfortunately, as there are no specific treatments available for DAI patients at this time, the value of improved imaging diagnosis currently lies in the potential to better prognosticate clinical outcome.52
Figure 8. Diffuse Axonal Injury in a 14 Year Old Following High Speed Motor Vehicle Accident. Axial NECT (a) Shows Two Hemorrhagic Foci in the Right Frontal Lobe. Axial GRE (b, c) MR Images Show Multiple Additional Foci of Susceptibility Within Bilateral Frontal Lobes, Predominantly Distributed Along the Gray-White Interface. In Addition, “Blooming” T2* Abnormality is Also Noted in the Splenium of Corpus Callosum
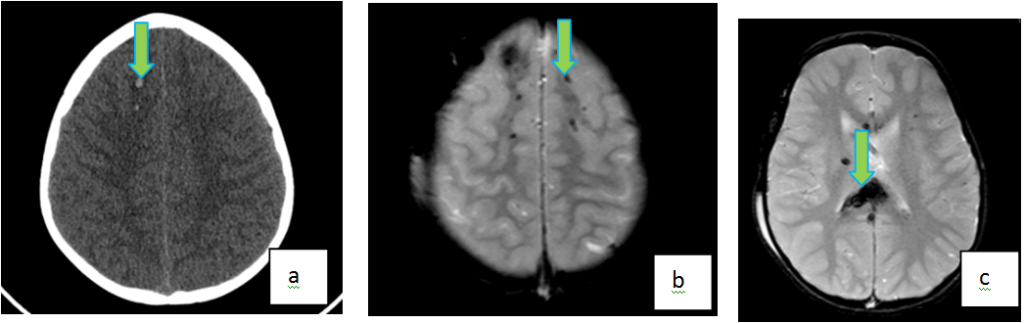
Age of the subacute and chronic TBI, MRI is recognized as the most appropriate initial examination. The latter may especially be important in cases of AHT, since MRI allows for better dating of intracranial abnormalities, as well as stratify the degree of injury, and thus, may be relevant in determining the temporal course of brain injury as seen on MRI (Table 1).
Challenges
One of the challenges of using MR in younger patients, particularly in those <6 years of age, is that these patients cannot remain still during the MRI exam, leading to motion artifact and poor image quality. Thus, MRI frequently necessitates use of sedation in these patients. In addition to the risks at the time of sedation, general anesthesia has the potential for causing developmental and behavioral problems in children. These risks are likely accentuated in patients with neurological injury. Therefore, rapid MRI sequences with shorter acquisition times are essential and have been developed to avoid this risk.53 Nevertheless, the implementation of such protocols widely will require evidence of improved outcomes from prospective studies. Clinicians need to balance the benefit of an MRI with the potential negative aspects, such as longer scan time and need for sedation.
CONCLUSION
To summarize, in the setting of head trauma, MRI provides an imaging modality with a unique ability to highlight critical information that CT and other imaging modalities cannot delineate. MRI has greater sensitivity in the detection of most types of head injuries, in comparison to CT – except skull fractures. In addition, MR techniques have the added benefit of having no ionizing radiation, thus decreasing radiation exposure and theoretically cancer risk as well. However, MRI has been a challenge to incorporate into the acute setting given the high-cost, relative lack of availability and highly skilled training for operating and prescribing sequences. Despite the challenges, MR imaging continues to play a growing role in the acute and sub-acute evaluation of pediatric head trauma, however, large, prospective clinical data will be needed to show evidence that MR as the initial imaging modality after pediatric head injury will allow for better short and long-term clinical outcomes than CT.
CONFLICTS OF INTEREST
The authors declare that they have no conflicts of interest.