INTRODUCTION
Ketoacidosis is sometimes a fatal complication of diabetes mellitus. Ketone bodies are typically produced in diabetic patients, but also by ketogenic diets and by fasting.
In the 1970’s the kinetics of ketone bodies were extensively studied after fasting and excersise.1,2,4,5,6 In the 1980’s various effects of hormones and fatty acids were reported.7-18 In the 1990’s βHB was found to be more than just a metabolite, having important cellular signaling roles.19,20,21,22,23 Notably, the inhibitory action on histone-demethylase opened prospects for therapeutic applications for various diseases. Recently ketone ester has been recommended as a dietary supplement for athletes.23
We have previously proposed the “dual engine” hypothesis to explain shifts in energy production: Basic ketone engine and booster glucose engine.24,25 However, individual differences in ketone production and the dynamics of ketogenesis are still unclear. Therefore, we tried to clarify the occurrence of ketogenesis during fasting by measuring concurrent changes in glucose, ketone bodies, fatty metabolism and various hormonal changes in both blood and urine. We recruited study subjects among participants in a comprehensive fasting program, which included a balanced diet, physical activity, and mind exercises to achieve a healthy spiritual life.
METHODS
Subjects
Fourteen healthy subjects admitted in the ARSOA Wellness Fasting program participated in the study between 10,11,12,13 September 2016.26 All participants gathered at the Relache Holistic Center in Megami-no-mori, Hokuto City, Yamanashi prefecture. Persons who wished to participate in the program were telephone-interviewed by an expert staff about their health and their willingness to join the program. Healthy adults of any age or gender could to participate in the program. We excluded pregnant women and patients receiving any medication for cardiovascular disease, diabetes, chronic infectious diseases, gastro-intestinal tract conditions, or cancer.
All participants signed a consent sheet after having received precise explanations about of the program. The program was approved by the Ethical Committee in the Life Science Promotion Association.
Program
The schedule and contents of wellness fasting program has been previously reported.26 During the 4-days fasting program, participants received a combination of very low-energy vegetarian diet (about 300 kcal per day intake from fermented vegetable juice), physical exercise (2-hours walking, slow training, and stretching), meditation, and lectures about healthy lifestyles (Figure 1).
Figure 1: Schema of the Wellness Fasting Program Thin Arrows Shows Drink Time (early morning, noon and evening) of Fermented Vegetable Juice and Mineral-Vitamin Supplement. Subjects Come to the Program without Taking Breakfast and Lunch on Day 1, and Leave after Taking Brown rice Brunch on day 4.
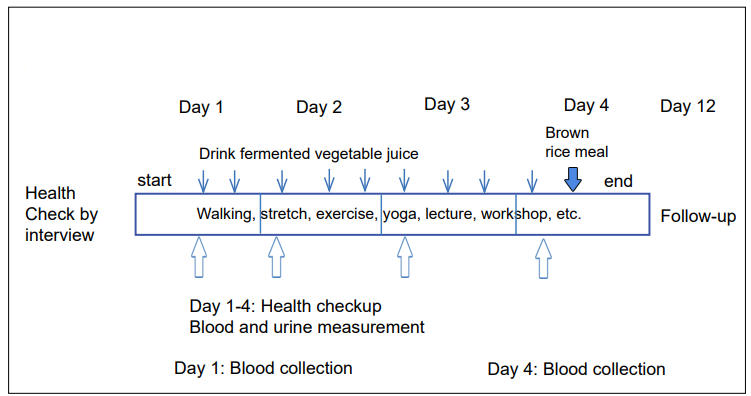
Every morning participants measured their body composition using the Body Composition Analyzer® (Tanita, Tokyo, Japan), which determines body weight, fat volume, muscle volume, water contents, estimated bone weight, basal metabolic rate and body mass index (BMI), based upon the electric impedance.27 Blood pressure and pulse rate were measured with the Omron HEM-7021® monitor (Kyoto, Japan).
Blood glucose and β-hydroxybutyrate (βHB) were measured every morning with the Freestyle Precision Neo® meter (Abott, Chicago, IL, USA).28 Daily first urine was checked for urinary pH, glucose, βHB, urobilinogen, proteins, occult blood, bilirubin, nitrate, and relative gravity using the Uropaper III® (Eiken, Tokyo, Japan). A sample of about 10 ml of urine was stored in a frozen tube for quantitative analysis of creatinine, acetoacetate (AcAc), βHB and catecholamines by the Serum Research Laboratory (SRL).29
All participants were requested to send urine at the 10th day after the fasting.
Laboratory Analyses
Sera at days 1 and 4 were quantitatively analyzed for ketone bodies (acetone, AcAc, βHB), insulin, glucagon, growth hormone, triiodo-thyronin, total thyroxin, triacyl-glycerol, free fatty acids, AST, ALT, γGTP and creatinine. The estimated glomerular filtration rate (eFGF) was calculated from age and creatinine value using the formula [male; eGFR (ml/min/1.73 m2 )=194×[age]-0.287×[CRE]-1.094, females; eGFR (ml/ min/1.73 m2 )=male_eGFR 0.739] recommended by the Japanese Renal Society.30
All urinary samples were quantitatively analyzed for creatinine, ketone bodies and catecholamines (epinephrine, norepinephrine and dopamine). Urine concentrations of these chemicals were standardized relative to creatinine levels.
Statistical Analysis
All data were reported in an Microsoft Excel® spreadsheet, and transferred to SPSS Version 22 (IBM SPSS, Tokyo, Japan). After double-checking all entries, aggregate data were expressed by mean and standard deviation (SD) if the distribution was normal. Otherwise, median and range were calculated, and nonparametric tests were used. The level of statistical significance was set at p<0.05. We also used Pass analysis to examine presumptive underlying variables by AMOS Version 22 for Windows (IBM Inc., New York, USA).31 The estimation of the best fitting model was carried out by the method of maximum likelihood of Pass analysis. Results were regarded as good model, if the RMSEA was less than 0.05.
RESULTS
The anthropometric data of subjects, basal metabolic rate (BMR) and body mass index (BMI) are shown in Table 1. Participants were 12 women and 2 men, ranging in age from 28 to 75. Sex differences in biochemical data were not apparent, so both genders were combined for statistical analysis. The magnitude of body weight loss after fasting was 2 kg on average. The rate of body fat consumption was variable, but visceral fat decreased especially among group 3 participants, if subjects were categorized by βHB concentration, as shown later. The systolic blood pressure decreased by 12 mmHg on average, and notably by 15 mmHg in diastolic pressure in group 3. On the contrary, the pulse rate increased. The body temperature decreased by 0.2- 0.4 o C. Subjective symptoms, such as headache, hunger, cold feeling, and gastro-intestinal distress were improved toward the last day of fasting.
Table 1: Anthropometric Data of Participants to 4-Day Wellness Fasting. |
Age (year) |
51.0±14.4 |
Height (cm) |
158.0±7.4 |
Weight (kg) |
54.9±10.7 |
Body fat percent (%) |
29.7±8.2 |
Water percent (%) |
49.5±4.4 |
Muscle_volume (kg) |
36.2±5.8 |
Water_weight (kg) |
27.0±4.9 |
Bone_weight (kg) |
2.1±0.4 |
BMR (kcal/day) |
1119±169 |
BMI (kg/m2) |
22.0±3.9 |
Ketone bodies in the blood increased from 0.3±0.2 mM at day 1 to 1.8±0.9 mM at day 4 of fasting, while glucose levels decreased from 5.8±1.9 mM to 4.5±1.5 mM on average (Figure 2). On day 4, in parallel with the increase in blood ketone bodies, the urinary excretion of AcAc increased to 40.9 mM (7.76- 159.2), βHB 25.1 mM (2.72-597.0) and total ketone bodies 66.1 mM (10.5-756.5) (Table 2). These deviations returned to 0.16 mM (0-10.1) after 14 days (10 days after fasting).
Figure 2: Individual Changes of Blood Glucose and β-hydroxybutyrate during 4-Day Fasting Subjects could be Divided to 3 Groups by Increase of BHB in the Blood.
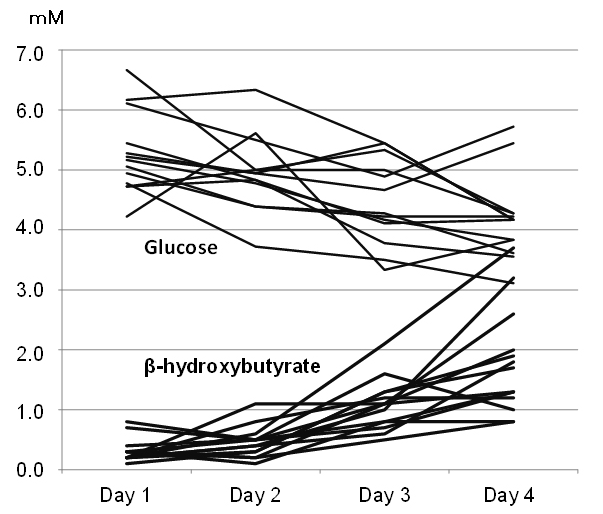
Table 2: Changes of Glucose and Ketone Bodies in the Blood and Urine. |
|
Day 1 |
Day 2 |
Day 3 |
Day 4 |
Day 14 |
Blood glucose (mM) |
5.8±1.9 |
5.1±0.7 |
4.8±1.3 |
4.5±1.5 |
|
Blood βHB (mM)1 |
0.3±0.2 |
0.5±0.3 |
1.1±0.4 |
1.8±0.9 |
|
Blood βHB (mM)2 |
0.17±0.17 |
|
|
2.0±1.0 |
|
Blood AcAc |
0.1±0.05 |
|
|
0.4±0.2 |
|
Urinary pH |
5.9±1.3 |
5.4±0.5 |
5.5±0.5 |
5.4±0.5 |
|
Urinary glucose |
– |
– |
– |
– |
– |
Urine AcAc (mM) |
0.1(0-29.8) |
1.2 (0.1-16.4) |
18.1 (2.0-46.3) |
40.9 (7.76-159.2) |
0.1 (0.02-6.2) |
Urine βHB (mM) |
0.1(0-15.1) |
0.6 (0.1-10.9) |
8.0 (1.0-116.2) |
25.1 (2.72-597.0) |
0.1 (0.06-3.85) |
Total Ketone bodies (mM) |
0.2 (0-44.9) |
1.0 (0.1-26.2) |
29.7 (3.2-192.1) |
66.1 (10.5-756.5) |
0.16 (0-10.1) |
Median (min-max) urinary ketone body concentration is standardized by urinary creatinine (g) 1 Abott precision; 2 Quantitative method |
The increase in βHB could be divided in 3 groups depending on the blood βHB level at the 4th day (0.8-1.2mM, 1.3-1.8 mM, 1.9-4.8 mM) (Table 3). The three groups showed different patterns in the production and urinary excretion of ketone bodies (Figure 3). The blood βHB/AcAc ratio was similar between the 3 groups, but the urine ratio was 0.56 in group 1, 0.75 in group 2 and 3.36 in group 3. The maximal excretion of βHB in the urine was 597.9 mmol/g creatinine. It was 100 times more of the blood βHB concentration.
Table 3: Pearson’s Correlation Coefficient between Selected Factors (Changes of Concentrations of Each Factors).
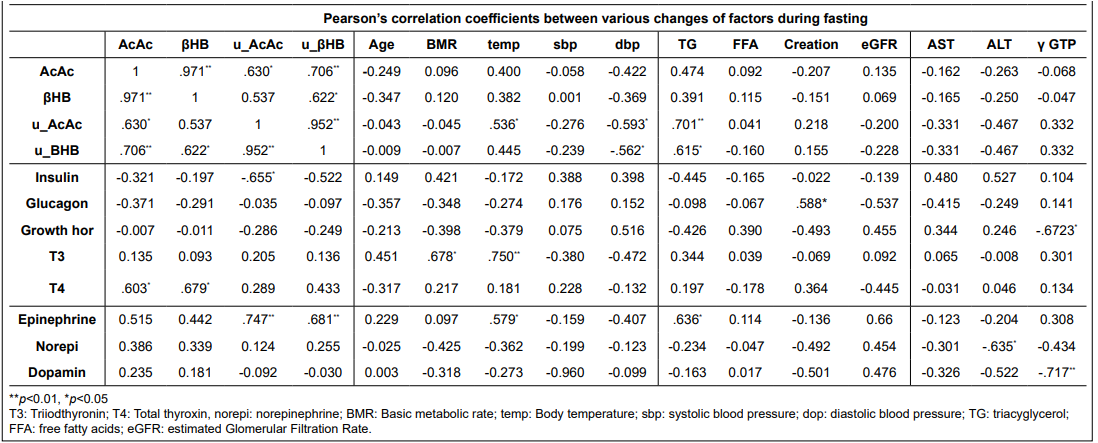
Figure 3: Changes of Serum βHB, AcAc and Urinary Ketone Bodies by the Group Category. Each Column Shows Values of Group 1, Group 2 and Group 3 from the Left. Urinary Excretion is 100 Times more of the Blood Ketone Bodies. In Group 3 Abrupt Excretion of βHB in the Urine is Noticed (rightest column). Box Plot Graph, Showing Median, 75-25 Percentile, and within 90 Percentile, and *is Outer Value of 90 Percentile.
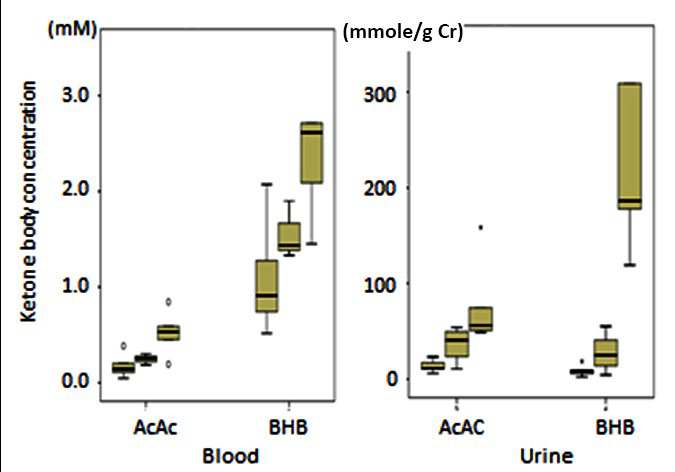
Decreases in insulin and inverse glucagon increases showed dose-dependent changes by groups. Decreased triacylglycerol and increased free fatty acids were largest in group 3. High ketosis in groups 2 and 3 were associated with low glucose level less than 4.5 Mm.
Growth hormone, tri-iodothyronin, and total thyroxin did not show significant change by groups, although total thyroxin (T4) correlated with blood ketone bodies, and urinary epinephrine significantly correlated with urinary ketone bodies (Table 3). AST and ALT slightly increased, while γGTP decreased in group 3.
Creatinine was slightly increased and the estimated glomerular filtration rate (eGFR) decreased by about 13% in all groups.
Pass analysis could resolve a complex relationship between the above described factors and the production of ketone bodies (Figure 4). Changes in insulin levels seemed to be the most effective factor to initiate an increase in βHB, followed by changes of BMR and body weight.
Figure 4: Pass Analysis. Changes of Insulin, BMR and Body Weight Significantly Contributed to the Increase of BHB and AcAc.
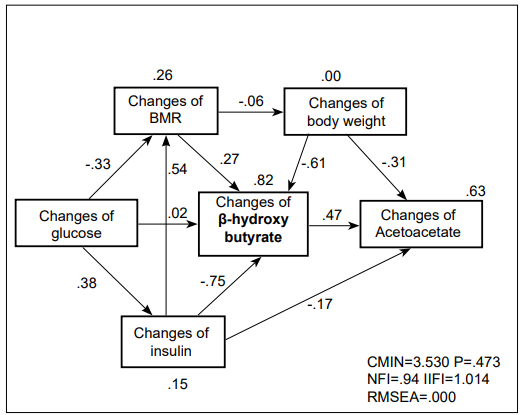
DISCUSSION
It has been shown that a few days of fasting causes many metabolic changes, involving various hormones and free fatty acid.32 Takahira et al33 was at the vanguard of fasting therapy in 1910-1920. He studied precisely the metabolic changes caused by fasting, and reviewed the efficacy of fasting therapy in various disease states. Mitsuo Koda34 developed fasting dietary therapy for various diseases, and confirmed beneficial effects for many patients with intractable diseases.
Cahill et al1 studied metabolic changes during 40 days of starvation and found that β-hydroxybutyrate replaced glucose as a source of energy. Fasting causes many metabolic changes,1,5,6,8 including a switch in cell fuel from glucose to fatty acids,24 but excretion of ketone bodies into the urine would cause energy loss.
The hyperglycemia and hyperketonemia of diabetic ketoacidosis are primarily initiated by the overproduction of ketone bodies, such as acetone, AcAc and βHB. Felty and Balasse13 demonstrated the production and disposal of ketone bodies in diabetic ketosis by using 14C-β-hydroxybutyrate or 14C-acetoacetate constant infusions in 13 insulin-dependent type-1 diabetic patients and 26 control subjects fasted for 15 hours to 23 days. Both groups showed a similar range of ketone body levels (1-12 mM) and turnover. The use of 14C isotopic methods is no longer possible now-a-days for ethical reasons, so their data should be considered the gold standard of ketone body turnover. They showed that ketone body production (a few mmol/min/1.73 m2 ) was correlated with plasma ketone body concentration, which reached a plateau after 3 days of fasting, and it was modified by the rate of metabolic disposal.
In their control group, ketonemia varied between 0.09 and 11.9 mM, and 22 subjects had total KB levels exceeding 2 mM with an average βHB/AcAc ratio 3.72±0.18. This group was similar to our group 3 subjects. They showed a curvilinear relationship between the metabolic clearance rate of total ketones and plasma ketone body concentration. A maximal metabolic disposal rate of about 2.3 mmole/min/1.73 m2 was attained in subjects for ketone concentrations exceeding 9 mM, corresponding to 331 mmole/day. The exponential pattern suggested that the removal capacities of tissues, such as muscle and brain, are progressively saturated as concentration rises. The hyperketonemia of fasting is primarily caused by an increased production of ketones, but the phenomenon is amplified by a progressive limitation in the ability of tissues to extract ketones from blood.
Keller et al17 using 14C-AcAc and Miles et al12 using 14C-βHB reported similar ketone production rates (about 1.2 mmole/min) in type-1 diabetic patients several hours after the interruption of an insulin infusion, but there was a more than threefold difference in the concentration of ketones, which averaged 2.2 mM in the Keller’s study and 7 mM in Miles’ study. It may be influenced by a faster removal of AcAc from blood to the tissue. Interestingly glucose homeostasis was very stable. Reichard et al20 estimated the average plasma glucose as 2.1 mM come from plasma acetone, and the proportion of 1,2-propanediol derived from plasma acetone as 74%. This should be needed for further confirmation.
Kellers et al11 reviewed the roles of insulin, catecholamines, and thyroid hormones in the regulation of ketone body kinetics. Insulin corrected diabetic ketoacidosis (DKA) largely via the suppression of lipolysis. In our study, inverse changes in insulin and glucagon were noteworthy. In addition, decreased triacylglycerol and increased free fatty acids appeared to be consumed for the production of ketone bodies by β-oxidation of fatty acids. Glucagon was said to be important in determining the magnitude of ketone body production for a given degree of FFA availability and insulin deficiency.10
On the contrary, the elevation of plasma glucagon during Intralipid® infusion failed to affect ketogenesis, and the βHB/AcAc concentration ratio decreased significantly (p<0.01).
In case of diabetic ketoacidosis, insulin administration is a effective therapy. Keller’s data indicate that the elevation of plasma insulin to high physiological concentrations restrains FFA-induced ketogenesis.16,17 Insulin administration lowers ketone body concentrations by three independent mechanisms: First, it inhibits lipolysis, and thus lowers free fatty acid availability for ketogenesis; second, it restrains ketone body production within the liver; third, it enhances the utilization of peripheral ketone body.19
As for the hormonal effects, thyroid hormones are said to regulate lipolysis, intrahepatic ketogenesis, and peripheral ketone body utilization,17 Norepinephrine has also been reported to increase ketogenesis, not only by stimulating lipolysis and releasing free fatty acids, but also by increasing intra-hepatic ketogenesis.18 In our study, changes of total thyroxin significantly correlated with blood ketone bodies, while changes of urinary epinephrine correlated with urinary ketone bodies, and dopamine showed significant decrease in group 3. Insufficient statistical power in our study could not clarify further effects of hormones, but the Pass analysis could suggest that decreased BMR and insulin significantly contributed to the increase in βHB and AcAc. A further larger study could clarify the above hormonal effects and kinetics of ketone bodies by a flux analysis.
Balasse et al13 observed that the blood concentrations, production rate and urinary elimination of total ketones after 3 days of fasting were 7.09±0.32 mM, 1.91±0.08 mmole/min, and 0.167±0.014 mmole/min, respectively. Urinary excretion was 24 mmol/day. These values correspond to our findings in group 2. The very high excretion rate of βHB in the urine in group 3 suggested that all produced βHB was excreted in the urine. This may cause renal damage as observed by a 13-14% decrease in eGFR in our study. Ketone bodies inhibit aminoacid metabolism and protein turnover in skeletal muscles, which may be an important survival mechanism during adaptation to catabolic states such as prolonged fasting.2,6
Risks and benefits of βHB should be further clarified, because slight liver and renal damages were suggested by increased AST, ALT, and a 12% decrease in eGFR.
Limitations of the present study is a small number of subjects that results in weak statistical power. We are now expanding the number of participants, and obtained nearly 60 subjects under the same protocol. We also try to analyze intestinal microbiota before and after fasting whether or not they contribute to the ketone body metabolism. The intention of this paper is to call attention about the efficacy and safety of fasting for raising ketone bodies for wellness and/or therapeutic aids.
CONCLUSION
Four days of fasting induces hyperketosis with some metabolic changes. The magnitude of changes in ketone body concentrations correlates with the levels of insulin, glucose, triacylglycerol and several hormones. The urinary excretion of βHB abruptly increased in group 3 (more than 1.8 mM). It was 100 times higher than the blood βHB concentration.
Four days of fasting induces hyperketosis with some metabolic changes. The magnitude of changes in ketone body concentrations correlates with the levels of insulin, glucose, triacylglycerol and several hormones. The urinary excretion of βHB abruptly increased in group 3 (more than 1.8 mM). It was 100 times higher than the blood βHB concentration.
ACKNOWLEDGEMENT
The authors thank participants and the staff of the wellness fasting program for their cooperation. We appreciate Dr. Philippe Calain for his constructive discussion and revising this manuscript.
CONFLICTS OF INTEREST
The study was supported by the ARSOA Educational Program.