Introduction
It is difficult to perform multiple cognitive demanding tasks concurrently, and multitasking could have a negative impact on memory and lead to negative outcomes in many fields, such as education, clinical care and in extreme work environments of the likes of air traffic control and crisis management. In the laboratory, the attentional blink (AB) paradigm1 has been widely used to understand multitasking limitations.2,3 In this dual-task paradigm, accurate report of a second target (T2) declines when presented within about half a second of a first target (T1) embedded in a rapid serial visual presentation (RSVP) of distractors. One important ongoing debate is whether the decline in T2 accuracy results from capacity-limitations in the consolidation of items into working memory, as postulated by capacity-based models,4,5,6,7 or rather from failures in attentional selection of items to be consolidated, as assumed by distractor-based models.
Whereas capacity-based models assume that the AB is caused by T1 processing, distractor-based models, such as Temporary Loss of Control (TLC),8,9,10 state that the AB is caused by interference of distractors during T1 processing. If a distractor is presented during consolidation of T1, it will disrupt the attentional filter set at the beginning of the trial to select targets and reject distractors optimally, leading to failures in temporal selection, which in turn can induce an increase in misselection errors.11,12,13,14,15,16,17
Contrary to TLC’s main claim, it has been shown that disruption of the attentional filter is not necessary to induce an AB, given that an AB can be observed in absence of intertarget distractors.18,19 However, intertarget distractors can directly modulate the AB without affecting T1 processing when no task switch is required between targets.20,21 This last result cannot be readily explained by capacity-based accounts, suggesting that the AB is not a unitary phenomenon,2 and that disruption of the attentional filter may play a role in modulating the AB in specific circumstances.
According to TLC, targets do not disrupt the attentional filter because they are compatible with the filter’s settings. This logic implies that distractors that are compatible with the filter’s settings should not disrupt the filter either, and consequently should not modulate the AB. The purpose of this study was two-folded. The first goal was to test this last assumption by manipulating distractor/filter compatibility as follows. Participants were required to identify two red uppercase letters embedded in a RSVP stream of distractors. In one block of trials, the distractor stream was composed of red digits. Given that the targets differed from the distractors only in respect to their category (letter targets and digit distractors), it was assumed that in this condition, a “category” filter would be established to select letters for – and/or reject digits from – entry into working memory. In the other block of trials, the distractor stream was composed of black letters. In this condition, distractors differed from the targets only in respect to their color (red targets and black distractors), and it was presumed that a “color” filter would be established to select red items – and/or reject black items. In other words, although the targets were defined by a conjunction of features (red and letter), only one of the two features was distinct from the distractor stream (either color in the black letter stream, or category in the red digit stream). Therefore, there is only one feature that can be used to select the targets. We hypothesized that the input filter would be configured in consequence (e.g., to let the red items through in the black letter stream or the letters through in the red digit streams).
In both blocks of trials, the distractor immediatly trailing T1 (the T1+1 distractor) could be a black digit, a black letter or a red digit. The black digit, which acts as a control, would be incompatible with the filter’s settings in both blocks of trials, because it differed from the targets in both category and color. The black letter would be compatible with the “category” filter established in the red digit stream block and incompatible with the “color” filter established in the black letter stream block. Inversely, the red digit would be compatible with the “color” filter established in the black letter stream block and incompatible with the “category” filter established in the red distractor stream condition. TLC would assume that incompatible T1+1 distractors would disrupt the filter, but not compatible T1+1 distractors.
If compatible distractors do not disrupt the filter, T2 accuracy would be lowest at Lag 3 (Lag refers to the number of items following T1), because it would be the T1+2 distractor that would disrupt the filter in these conditions instead of the T1+1 distractor. Furthermore, T2 accuracy should increase from Lags 3 to 9 in compatible conditions, and should be equivalent at Lag 9 and Lag 2, indicating an absence of AB at this shortest Lag, a phenomenon that will be termed Lag-2 sparing, based on terminology used in previous studies22 to provide a link with Lag-1 sparing, which refers to the well-known absence of a T2 deficit when no task or spatial shift occurs between targets appearing within about 150 msec from each other.23
The second goal was to determine whether the T1+1 distractor was mistaken for targets more often when it was compatible with the filter’s setting than when it was incompatible. If this were the case, it would provide novel insights as to the role of the input filter in misselection during the AB.11,12,13,14,15,16,17 To anticipate results, we observed that misselection of the T1+1 distractor was strongly modulated when it could be mistaken as a target (when T1+1 was of the same response category as the targets) and was compatible with the input filter’s settings. A follow-up experiment was therefore conducted to test whether this increase in T1+1 misselection errors was caused by the compatibility of the T1+1 distractor with the input filter’s setting or by a confounding factor, namely the novelty/salience of the T1+1 distractor in the compatible condition. In this follow-up experiment, novelty/salience was manipulated in conditions where the T1+1 distractor was always incompatible with the filter’s settings and was always of the same response category as the targets. This was accomplished by presenting red target letters and a black or blue T1+1 distractor letter within a distractor stream that was composed of either black or blue letters.
METHODS
Participants
Sixteen undergraduate students at the Université du Québec à Trois-Rivières participated in each experiment for financial compensation. All reported normal or corrected-to normal vision and were naive to the purpose of the experiment. The appropriate ethics committee at the Université du Québec à Trois-Rivières vetted the study protocol.
Apparatus and Stimuli
The experiments were programmed in E-Prime 2.0 and were run on a Pentium PC computer, with a 16-in CRT monitor with a refresh rate of 60 Hz. The RSVP stream, presented in the center of the screen, consisted of items presented in 20-point bold Courier New font, on a white background. Targets were red uppercase letters chosen randomly from the English alphabet with the exception of B, I, O, Q, Y, and Z, with the constraint that the identities of both targets were different. There were two distractor stream conditions in the main experiment (Experiment 1A). In the red digit distractor stream condition, the distractor stream consisted of red digits selected randomly, with replacement, from the set of digits 2-9, with the constraint that the selected digit was not one of the two preceding items. In the black letter distractor stream condition, a subset of eight uppercase letters were chosen before each trial from the English alphabet with the exception of B, I, O, Q, Y, Z, the letters chosen as T1, as T1+1 (when T1+1 was a letter) and as T2. Letters from this subset were selected randomly, with replacement, with the constraint that the selected letter was not one of the two preceding items. In both distractor stream conditions, the distractors immediately trailing T1 (T1+1 distractor) could be a black digit, a black letter, or a red digit. The identity of the T1+1 distractor was never the same as the identity of either targets, nor as the identity of the other distractors in the stream.
Each item in the RSVP stream remained on the screen for 100 msec, and was immediately followed by the next item without any inter-stimulus interval (ISI), yielding a presentation rate of 10 items/sec. The number of distractors preceding T1 was determined randomly on each trial and varied between 6 and 12. T2 was presented either at Lag 2, at Lag 3, at Lag 4 or at Lag 9, and was followed by only one distractor that acted as a mask.
In the follow-up experiment (Experiment 1B), the stimuli were identical, with the exception that the distractor streams were composed of either blue uppercase letters or black uppercase letters and the T1+1 distractor was either a blue letter or a black letter (Figure 1).
Figure 1: Illustration of the RSVP streams used in Experiment 1A for both distractor stream conditions at the T1-T2 Lag 2. Two separate unspeeded responses were required on each trial.
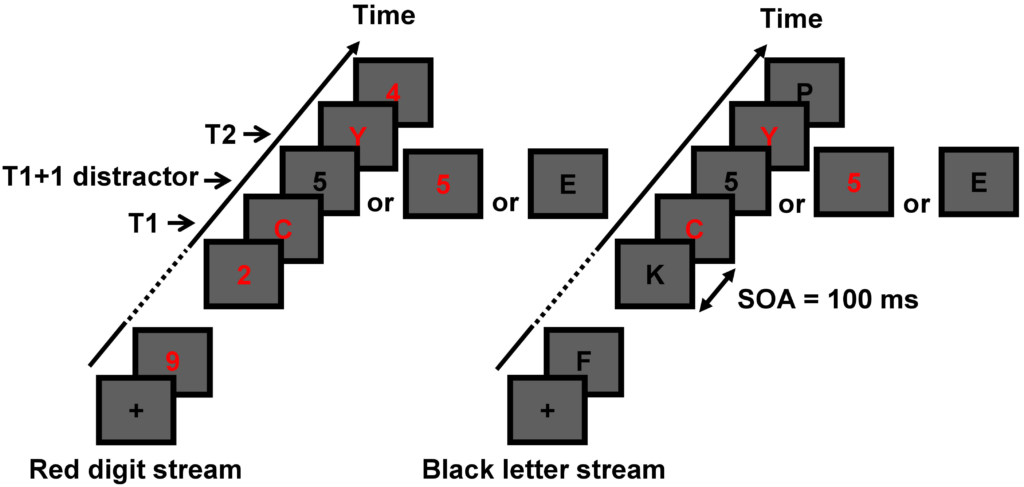
Design and Procedure
Each participant took part in one experimental session. Experiment 1A consisted of two experimental blocks of 240 trials, each preceded by one practice block of 12 trials. Distractor stream conditions were blocked (order counterbalanced between subjects). T1+1 distractor and T1-T2 Lag conditions were randomly intermixed in each block of trials. Experiment 1B consisted of two experimental blocks of 160 trials, each preceded by one practice block of 8 trials. Distractor stream conditions were blocked (order counterbalanced between subjects). T1+1 distractor and T1-T2 Lag conditions were randomly intermixed in each block of trials.
For both experiments, participants were required to read the instructions displayed on the screen at the beginning of the session, and were invited to ask questions to clarify procedural points if needed. Participants were required to report, without speed pressure, the identity of the red letters (T1 and T2), by pressing the corresponding key on the keyboard when prompted.
Each trial began with a small black fixation cross presented in the center of the screen, indicating the location at which the RSVP stream was about to appear. Participants initiated each trial by pressing the space bar. The RSVP stream started 200-500 msec after trial initiation. Two hundred to five hundred msec after the stream ended, a first question, “Quelle était la première lettre rouge [French, meaning: What was the first red letter?]”, was displayed until response. A second question, “Quelle était la seconde lettre rouge [French, meaning: What was the second red letter?]”, was displayed immediately after the first response, until the second response was executed, at which point the fixation cross reappeared, indicating that the participant could initiate the next trial when desired.
RESULTS
The instructions in both experiments explicitly informed participants to respond to targets in order of appearance. Consequently, temporal order of report of targets was taken into account when scoring the accuracy of the responses (i.e., T1 was scored as correct only if it was reported as T1, and T2 was scored as correct only if it was reported as T2). A Greenhouse-Geisser procedure was applied when appropriate to counter violations of the sphericity assumption.
Experiment 1A
T1 performance: Mean accuracy for T1 is plotted as a function of T1+1 distractor and T1-T2 Lag in Figure 2 for the red digit distractor stream (upper left panel) and for the black letter distractor stream conditions (upper right panel). Mean accuracy of T1 was submitted to a repeated measures analysis of variance (ANOVA) in which distractor stream, (2 levels: red digit stream and black letter stream), T1+1 distractor (3 levels: compatible, incompatible, control), and T1-T2 Lag (4 levels: Lag 2, Lag 3, Lag 4, Lag 9), were included as within-subject factors.Footnote[1]
Figure 2: Results from Experiment 1A. Mean accuracy for T1 (first target) is plotted as a function of T1+1 distractor and T1-T2 Lag for the red digit distractor stream condition in the upper left panel and for the black letter distractor stream condition in the upper right panel. Mean accuracy for T2 (second target) in trials where T1 was correctly reported (T2|T1) is plotted as a function of T1+1 distractor and T1-T2 Lag for the red digit distractor stream condition in the lower left panel and for the black letter distractor stream condition in the lower right panel. Error bars represent standard error of the mean.
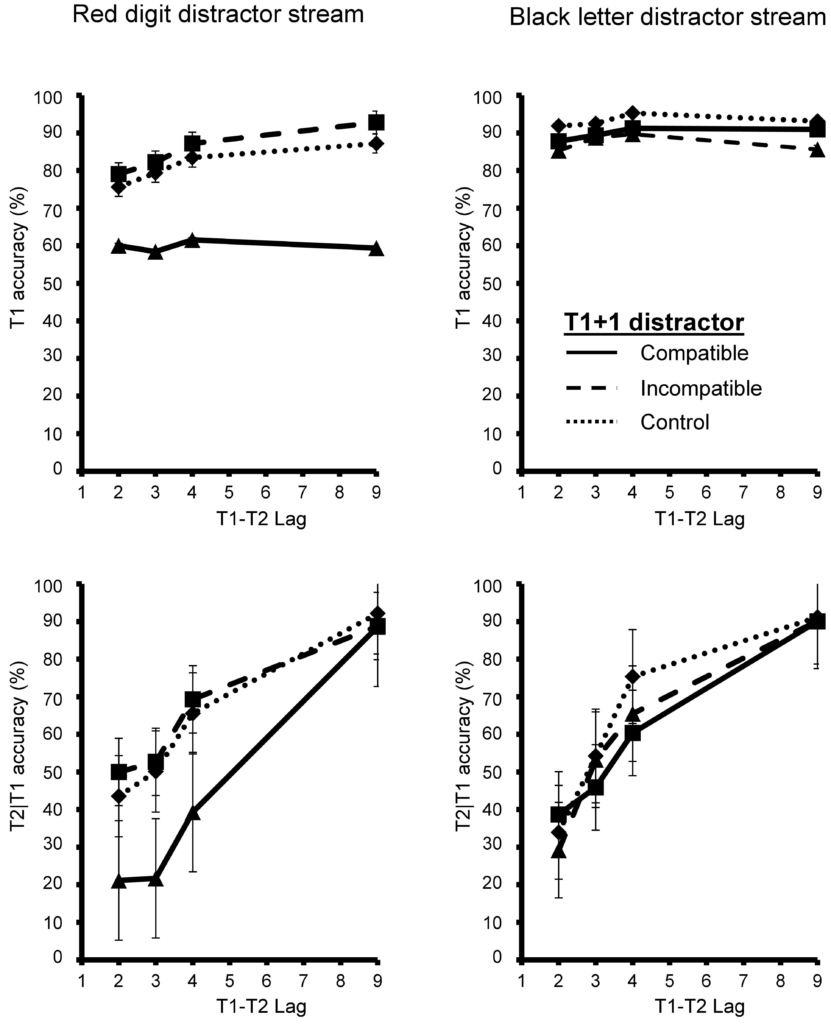
Footnote
[1] A preliminary analysis including block order as a between-subject factor in mixed ANOVAs were performed for both T1 and T2 performance. No main effect or interactions with this factor were significant when analyzing T1 performance. No main effect or interactions with this factor were significant when analyzing T2 performance, except for the block order × distractor stream interaction, F(1, 14)=8.85; ηp2=.387; p<.05, which revealed practice effects. Block order was therefore excluded from further analysis.
The distractor stream × T1+1 distractor × Lag interaction was not significant, F(6, 90)=1.86; ηp2=.110; p>.05, nor was the T1+1 distractor × Lag interaction, F<1. However, a distractor stream × Lag interaction was observed, F(3, 45)=3.41; ηp2=.185; p<.05, as well as a distractor stream × T1+1 distractor interaction, F(2, 28)=63.12; ηp2=.808, p<.05. To understand these significant interactions, subsequent analyses were performed for each distractor stream condition.
In the red digit stream condition, a main effect of Lag was present, F(3, 45)=7.55; ηp2=.335; p<.05, with T1 accuracy increasing progressively with Lag. The main effect of T1+1 distractor was also significant, F(2, 30)=78.02; ηp2=.839; p<.05. The T1+1 condition × Lag interaction, on the other hand, was not significant F(6, 90)=1.87; ηp2=.111; p>.05. Scheffé’s contrast procedure24,25 showed that T1 accuracy was lower in the compatible condition (mean T1 accuracy=60%) than in the incompatible (mean T1 accuracy=85%) and in the control conditions (mean T1 accuracy=81%, FScheffé(2, 30)=149.80; p<.10).Footnote[2] No significant difference was observed between the two latter25 conditions, FScheffé(2, 30)=3.09; p>.10.
Footnote
[2] As proposed by Scheffé, this test was run at α=.10, to overcome its low power.26
In the black letter stream condition, the main effect of Lag was not significant, F(3, 45)=1.87; ηp2=.111; p>.05, nor was the T1+1 condition × Lag interaction, F<1. However, a main effect of T1+1 distractor was observed, F(2, 30)=10.44; ηp2=.410; p<.05. Scheffé’s contrast procedure showed that T1 accuracy was slightly higher in the control condition (mean T1 accuracy=93%) than in the compatible (mean T1 accuracy=90%, FScheffé (2, 30)=6.74; p<.10) and in the incompatible condition (mean T1 accuracy=87%, FScheffé(2, 30)=20.50; p<.10) . No significant difference was observed between the two latter conditions,
FScheffé(2, 30)=3.73; p>.10.
T1 misselection errors: To measure T1 misselection errors, we first selected the trials in which T1 was incorrectly reported, using only trials where the T1+1 distractor was a black letter (which are the only trials in which the T1+1 distractor was of the same response category as the targets). We then calculated the percentage of these trials where the T1+1 distractor was mistaken for T1 (Figure 3, left panel). Finally, the data were submitted to a repeated measures ANOVA with T1+1 compatibility (2 levels: compatible and incompatible) and T1-T2 Lag (4 levels: Lag 2, Lag 3, Lag 4, Lag 9), as within-subject factors. Six participants were excluded from the analysis because they had no T1 incorrect trials in at least one T1+1 distractor × Lag cell. A main effect of T1+1 compatibility was observed, F(1, 9)=8.79; ηp2=.494; p<.05, indicating that participants mistook the T1+1 distractor for T1 more often in the compatible condition (63%) than the incompatible condition (47%). The main effect of Lag was not significant, F(3, 27)=1.92; ηp2=.176; p>.05, nor was the interaction between the two factors, F<1.
Figure 3: Results from Experiment 1A. Percentage of T1 misselection errors in incorrectly reported T1 (left panel) and percentage of T2 misselection errors in correctly reported T1 and incorrectly reported T2 trials (right panel) when the T1+1 distractor was a black letter, plotted as a function of T1-T2 Lag and T1+1 compatibility conditions. Error bars represent standard error of the mean.
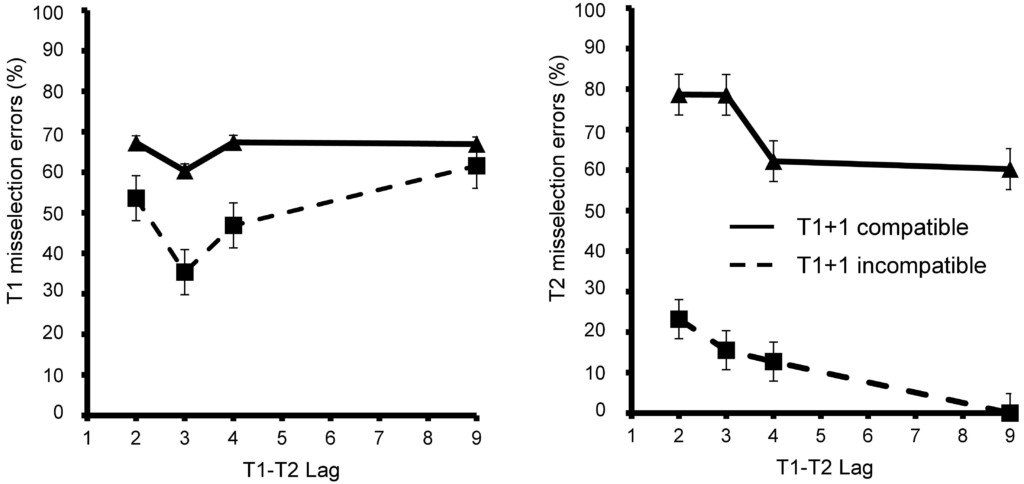
T2|T1 performance: Mean accuracy for T2|T1 is plotted as a function of T1+1 distractor and T1-T2 Lag in Figure 2 for the red digit distractor stream (lower left panel) and for the black letter distractor stream conditions (lower right panel). Mean accuracy of T2|T1 was also submitted to a 2×3×4 repeated measures ANOVA with distractor stream, T1+1 distractor and T1-T2 Lag as within-subject factors.
A triple interaction was observed, F(6, 90)=5.04; ηp2=.251; p<.05. To understand this interaction, subsequent analyses were performed for each distractor stream condition. In the red digit stream condition, the main effect of Lag was significant, F(3, 45)=61.40; ηp2=.804; p<.05, showing that T2 accuracy increased from Lag 2 to Lag 9, which indicates that an AB was observed. The main effect of T1+1 distractor was also significant, F(2, 30)=43.76; ηp2=.745; p<.05. Scheffé’s contrast procedure showed that T2 accuracy was lower in the compatible condition (mean T2 accuracy=43%) than in the incompatible (mean T2 accuracy=65%) and in the control condition (mean T2 accuracy=63%; FScheffé(2, 30)=77.68; p<.10). No significant difference was observed between the two latter conditions, FScheffé<1. The T1+1 distractor × Lag interaction was also significant, F(6, 90)=7.66; ηp2=.338; p<.05. Scheffé’s contrast procedure indicated that the AB (calculated as Lag 9 minus Lag 2) was larger in the compatible condition (mean AB: 68%) compared to the incompatible condition (mean AB: 39%), and the control condition (mean AB: 49%), FScheffé(2, 30)=28.96; p<.10. No significant difference was observed between the two latter conditions, FScheffé(2, 30)=3.69; p>.10.
In the black letter stream condition, the main effect of Lag was still significant, F(3, 45)=57.40; ηp2=.793; p<.05. However, the main effect of T1+1 distractor was not, F(2, 30)=2.40; ηp2=.138; p>.05. Although a T1+1 distractor × Lag interaction was observed, F(6, 90)=3.53; ηp2=.190; p<.05, Scheffé’s contrast procedure revealed only a marginally significant attenuation of the AB in the compatible condition (mean AB: 51%) compared to the control condition (mean AB: 57%) and the incompatible condition (mean AB:61%), FScheffé(2, 30)=3.89; p>.10, and no significant difference between the two latter conditions,
FScheffé<1.
To investigate whether the T1+1 distractor × Lag interactions observed above were the consequence of the compatibility of the T1+1 distractor with the input filter, or the result of distractors category (digit or letter), we performed a repeated measures ANOVA for each T1+1 category (digit and letter), with T1+1 compatibility (2 levels: compatible and incompatible) and Lag (4 levels: Lag 2, Lag 3, Lag 4, and Lag 5) as within subject factors. If compatibility of the T1+1 distractor with the input filter drove the above interactions, then we should observe a T1+1 compatibility × Lag interaction for both T1+1 categories.
When the T1+1 distractor was a letter, a T1 compatibility × Lag interaction was observed, F(3, 45)=7.90; ηp2=.345; p<.05, as well as a main effect of T1 compatibility, F(1, 15)=14.07; ηp2=.484; p<.05. However, when the T1+1 distractor was a digit, the T1 compatibility × Lag interaction was not significant, F(3, 45)=1.64; ηp2=.099; p>.05, nor was the main effect of T1 compatibility effect, F(1,15)=3.30; ηp2=.180; p>.05.
T2 misselection errors: To measure T2 misselection errors in T1 correct trials, we first selected the trials in which T1 was correctly reported but T2 was not correctly reported, using only trials where the T1+1 distractor was a black letter. We then calculated the percentage of these trials where the T1+1 distractor was mistaken for T2 (Figure 3, right panel). Finally, the data were submitted to a repeated measures ANOVA with T1+1 compatibility (2 levels: compatible and incompatible) and T1-T2 Lag (4 levels: Lag 2, Lag 3, Lag 4, Lag 9), as within-subject factors. Eight participants were excluded from the analysis because they had no T2 incorrect trials in at least one T1+1 distractor × Lag cell. A main effect of T1+1 compatibility was observed, F(1, 7)=71.49; ηp2=.911; p<.05, indicating that participants mistook the T1+1 distractor for T2 more often in the compatible condition (70%) than the incompatible condition (14%). The main effect of Lag was not significant, F(3, 21)=2.10; ηp2=.231; p>.05, nor was the interaction between the two factors, F<1.
Experiment 1B
T1 performance: Mean accuracy for T1 is plotted as a function of T1+1 distractor and T1-T2 Lag in Figure 4 for the blue letter distractor stream (upper left panel) and for the black letter distractor stream conditions (upper right panel). Mean accuracy of T1 was submitted to an ANOVA in which distractors stream (2 levels: blue letter stream and black letter stream), T1+1 distractor (2 levels: same color and different color), and T1-T2 Lag (4 levels: Lag 2, Lag 3, Lag 4, Lag 9), were included as within-subject factors. No main effects or interactions were observed (all Fs<1.7, except the main effect of Lag, F(3, 45)=2.33; ηp2=.134; p>.05).
Figure 4: Results from Experiment 1B. Mean accuracy for T1 is plotted as a function of T1+1 distractor and T1-T2 Lag for the blue letter distractor stream in the upper left panel and for the black letter distractor stream conditions in the upper right panel. Mean accuracy for T2 in trials where T1 was correctly reported (T2|T1) is plotted as a function of T1+1 distractor and T1-T2 Lag for the blue letter distractor stream condition in the lower left panel and for the black letter distractor stream condition in the lower right panel. Error bars represent standard error of the mean.
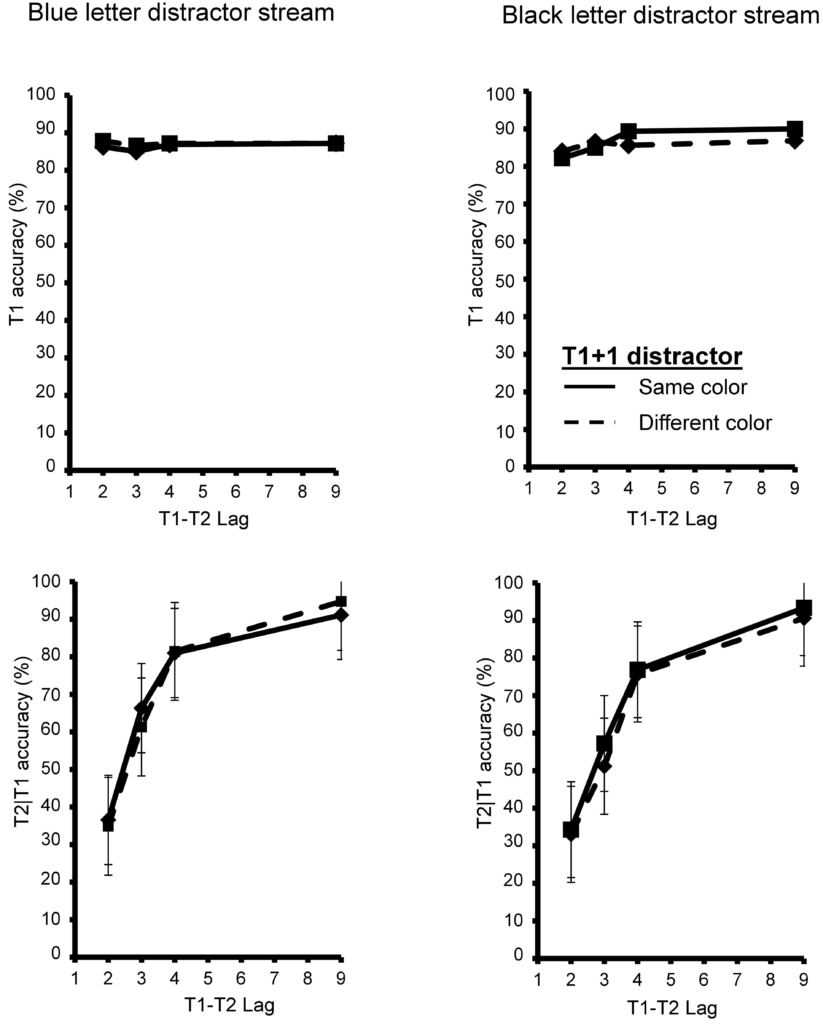
T1 misselection errors: The percentage of T1 misselection errors is plotted as a function of T1+1 distractor conditions, T1-T2 Lag and distractor stream in Figure 5 (upper panels). A repeated measures ANOVA was performed, with distractors stream (2 levels: blue letter stream and black letter stream), T1+1 distractor (2 levels: same color and different color), and T1-T2 Lag (4 levels: Lag 2, Lag 3, Lag 4, Lag 9) as within-subject factors. Eight participants were excluded from the analysis because they had no T1 incorrect trials in at least one T1+1 distractor × Lag cell. No main effects or interactions were observed (all Fs<2.03, all ps>.17). Note that the absence of any effects in this analysis could be the result of the large number of excluded participants combined with the low number of incorrect T1 trials in Experiment 1B.
Figure 5: Results from Experiment 1B. Percentage of T1 misselection errors in incorrect T1 trials (upper panels) and of T2 misselection errors in correctly reported T1 and incorrectly reported T2 trials (lower panels), plotted as a function of T1+1 distractor and T1-T2 Lag for the blue letter distractor stream condition (left panels) and for the black letter distractor stream condition (right panels). Error bars represent standard error of
the mean.
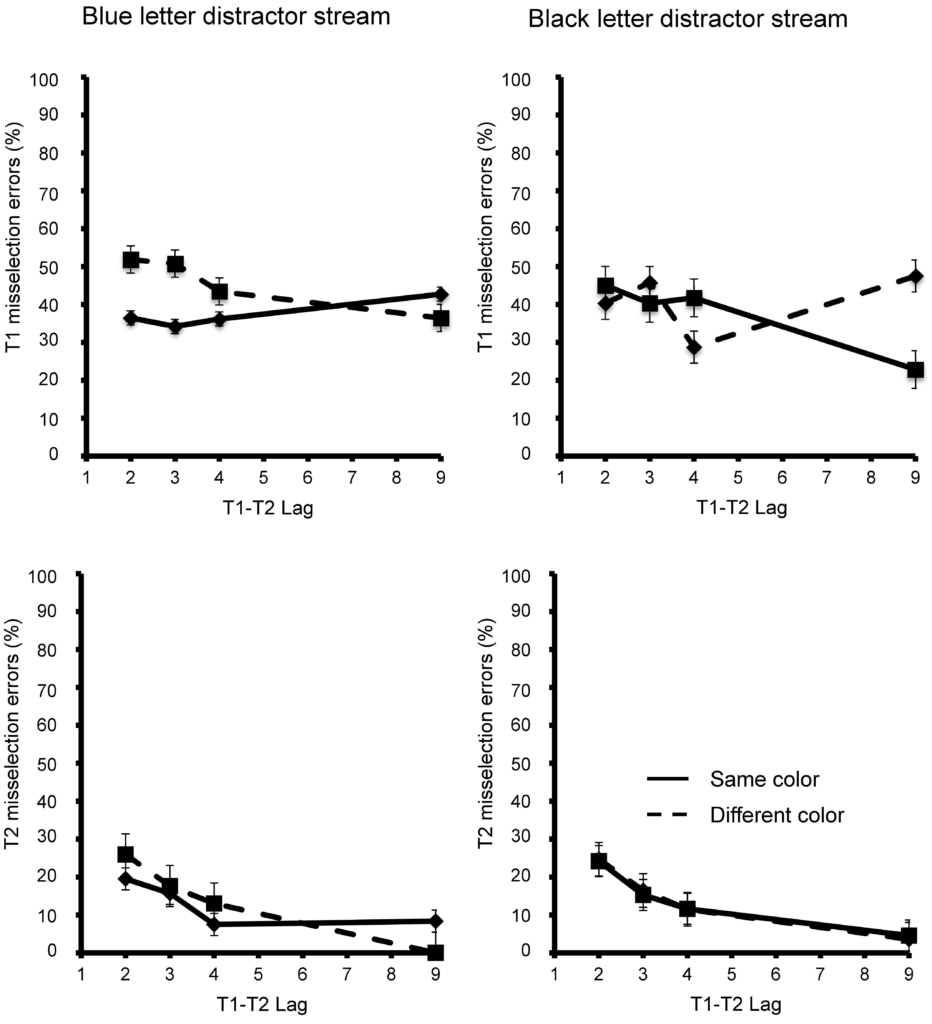
T2|T1 performance: Mean accuracy for T2|T1 is plotted as a function of T1+1 distractor and T1-T2 Lag in Figure 4 for the blue letter distractor stream (lower left panel) and for the black letter distractor stream conditions (lower right panel). Mean accuracy of T2|T1 was also submitted to a repeated measures ANOVA with distractors stream (2 levels: blue letter stream and black letter stream), T1+1 distractor (2 levels: same color and different color), and T1-T2 Lag (4 levels: Lag 2, Lag 3, Lag 4, Lag 9) as within-subject factors. As in Experiment 1A, T2|T1 performance was lowest at the shortest Lag and increased as Lag increased, leading to a main effect of Lag, F(3, 45)=62.64; ηp2=.807; p<.05.The main effect of distractor stream was not significant, F(1,15)=1.52; ηp2=.092; p>.05, nor was the interaction between distractor stream and Lag, F(3,45)=1.24; ηp2=.076; p<.05. Importantly for the purpose of Experiment 1B, the main effect of T1+1 distractor was not significant, F(1,15)=1.52; ηp2=.092; p>.05, nor was the interaction between T1+1 distractor and distractor stream, F<1.
T2 misselection errors: The percentage of T2 misselection errors is plotted as a function of T1+1 distractor conditions, T1-T2 Lag and distractor stream in Figure 5 (lower panels). A repeated measures ANOVA was performed, with distractors stream (2 levels: blue letter stream and black letter stream), T1+1 distractor (2 levels: same color and different color), and T1-T2 Lag (4 levels: Lag 2, Lag 3, Lag 4, Lag 9) as within-subject factors. Ten participants were excluded from the analysis because they had no T2 incorrect trials in at least one T1+1 distractor × Lag cell. A main effect of Lag was observed, F(3,15)=6.27; ηp2=.556; p<.05, indicating that T2 misselection occurred more often at shorter than longer T1-T2 Lags. All other main effects and interactions were not significant (all Fs<1), indicating that novelty had no impact on misselection of the T1+1 distractor.
DISCUSSION
The present study was conducted to investigate how compatibility of the distractor immediately trailing T1 (T1+1 distractor) with the attentional filter’s settings affects T2 performance and misselection errors in the AB.
Investigating distractor/filter compatibility is particularly important to evaluate the Temporary Loss of Control (TLC) theory.8,9,10 TLC is a distractor-based theory that assumes that distractors presented between targets (known as intervening, or intertarget distractors) are the root cause of the AB. These theories have been challenged by the observation that an AB can be observed in absence of intertarget distractors.18,21,27 Although intertarget distractors may not be necessary to observe an AB, they can directly modulate the effect when no task switch is required between targets,20,21 suggesting that distractor-based interference may nevertheless play an important role in the deficit.
TLC predicts that compatible T1+1 distractors should not disrupt the attentional filter’s settings and consequently should spare the following item in the RSVP stream from the AB deficit. In other words, according to TLC, we should observe Lag-2 sparing in the compatible T1+1 conditions in the same manner as the third of three successive targets is spared from an AB.8 Contrary to this prediction, we did not observe any effect of T1+1 distractor compatibility on the AB, when the T1+1 distractor was a digit.
The absence of Lag-2 sparing is in opposition to a previous study that used a similar manipulation.15 In this study, participants had to identify two red letter targets embedded in a black digit stream of distractors. There were three main conditions: (1) a standard condition where all distractors were black, (2) a T1+1 red condition where the T1+1 distractor was red, and (3) a T1+2 red condition where the T1+1 and T1+2 red distractors were red. Although sparing was observed in the T1+1 and T1+2 red distractor conditions, this effect may have been the results of cueing, which is known to attenuate the AB.14,28 Indeed, in the task designed by Olivers and Meeter, targets differed from distractors along two features (color and category). Given that color facilitated – but was not required for – target selection, participants could perform the task successfully, in all conditions, by using a category-filter. This is a critical difference with the current study, where targets differed from distractors in only one feature (color or category) in most conditions, forcing participants to implement either a color-filter or a category-filter.
The absence of a compatibility effect with digit T1+1 distractors was the first evidence against TLC. When the T1+1 distractor was a letter, and therefore could be mistaken as a target, a compatibility effect was observed, but in the opposite direction as TLC predicted. These results are hard to reconcile with the TLC framework. Furthermore, the last results supports an important assumption underlying our design, mainly that participants would adopt a “red” color-filter in the black letter distractor stream and a “letter” category-filter in the red digit distractor stream condition, rather than a more precise “red letter” filter in both distractor stream conditions. If participants would have adopted a “red letter” filter in all conditions, than all types of T1+1 distractors would have been incompatible with all filters, and therefore no effect of T1+1 compatibility would have been observed. Given the effect of T1+1 compatibility observed in the red digit stream condition of the main experiment (Experiment 1A), we can be rather confident that our initial assumption is valid, and that the observed results cannot be explained simply by an inefficient manipulation of T1+1 distractor compatibility. But what could explain the increase in AB amplitude in the T1+1 compatible condition when the T1+1 distractor was a letter?
Interestingly, it could be explained by an increase in misselection errors. Specifically, when the T1+1 distractor was a letter, participants tended to misselect this item as targets. T2 misselection occurred three-to-four times more often when it was compatible with the filter’s settings than when it was not. Although more distractors could be mistaken for targets in the black letter stream than in the red digit stream, this could not explain the whole of the effect. Indeed, items that are misselected tend to be the items immediately preceding or trailing the targets.17,29 Consequently, in trials where T1 was correctly identified and misselection occurred, the misselected item would be either the distractor immediately trailing or immediately leading T2, which would double the probability of misselection that was observed in the black letter stream. However, the main effect of T1+1 compatibility was still observed when doubling- and even tripling- the probability of misselection in the black letter stream.Footnote[3] Note that the increase in misselection errors also suggests that category was effectively filtered while color was mostly ignored in the red digit stream trials, increasing the probability that a black letter would be reported as a target.
Footnote
[3] Tripling the probability of misselection led to a compability x Lag interaction, indicating that a compatibility effect was observed at all Lags, except Lag 2.
T1 performance was also lowest in the compatible red digit distractor condition, which could be an indication that the T1+1 distractor in this condition increased T1 difficulty in correctly reported trials, which in turn produced a larger blink.19,30,31 However, misselection of T1 was also greater in this condition. If we considered T1 misselection trials as correct T1 trials, no main effect of T1+1 distractor or interaction with this factor was observed. This suggests that T1 processing was not more difficult in the compatible red digit stream condition when T1 was correctly selected and reported.
It is important to notice however that in the main experiment, the T1+1 letter distractor was salient and novel in the compatible condition (the only black item in a red distractor stream), whereas it was similar to the other distractors in the incompatible condition (one of several black letters in a black letter distractor stream). The distinctiveness of the T1+1 distractor in the compatible condition could have exogenously captured attention32,33 and hence increased its likelihood of entering working memory for further report. However, the follow-up experiment (Experiment 1B) provided evidence against this hypothesis. Indeed, results showed that novelty/salience of the T1+1 distractors does not modulate the AB nor the number of T2 or T1 misselection errors when the distractor is incompatible with the filter’s settings. Some theories of attentional capture, such as the attentional control theory,34 suggest that compatibility with the filter’s settings inherently increases saliency of compatible items, leading to greater attentional capture. It may also be proposed that the novel item was not as salient here than in the former experiment, given that targets were also of a different color than the distractor stream in the present experiment, but not in the red digit stream of the main experiment. Nevertheless, the results of the follow-up experiment support the view that compatibility of the T1+1 distractor with the input filter’s settings is a critical factor driving the increase in misselection errors observed in the main experiment. Again, this conclusion is consistent with the attentional capture literature, which proposes that attentional capture only occurs when the item matches the attentional control settings.35 This conclusion is also in line with the absence of an effect in the control T1+1 distractor condition of the red distractor stream of the main experiment, where the T1+1 distractor was salient, but was not compatible with the filter’s setting.
It has recently been suggested that features from consecutive items may merge in conditions leading to Lag-1 sparing.36 It is therefore possible that the identity of the T1+1 distractor letter may have combined with the red color of T1 in some of the compatible red digit stream trials. This could explain the increase in T1 misselection errors in these trials. However, it cannot explain the large increase in T2 misselection errors at all Lags when T1 was correctly reported, since it would only predict an increase in T2 misselection errors at Lag 2, which is the only Lag where T2 immediately follows the T1+1 distractor.
The present results support the claim that misselection may play an important role in the AB.14,17,28 However, contrary to many of the previous studies which have observed a propensity of T2+1 misselection errors in the AB,11,16,17,29,37 here we highlight T1+1 misselection errors on both T1 and T2, and show that these types of errors are highly dependent on the relationship between the misselected item and the settings of the input filter.
Although T1+1 distractor misselection modulated the AB when the misselected item was compatible with the attentional filter’s settings and was of the same response category as the target, and AB was observed in all conditions, which indicates that although misselection can modulate the AB significantly, it is not necessarily the main cause of the AB. In fact, the present results support the view that the AB is not a unitary phenomenon and that different AB tasks reflect distinct processing limitations,20,38 which could include task-switching,23 capacity-limitations in working memory consolidation4,5,39 and/or distractor-based interference that impacts on target selection processes.11,13,16,21
In summary, the present study provides strong evidence against one key assumption of the TLC framework, which states that items that are compatible with the input filter’s settings will not elicit an AB, by showing that T1+1 distractors that are compatible with the input filter’s settings do not lead to Lag-2 sparing. However, we show that compatibility of the T1+1 distractor with the input filter can modulate the AB by varying T1+1 distractor misselection errors, which increases dramatically in compatible conditions.
AKNOWLEGEMENT
This research was supported by Discovery (402614-2011) and Research Tools and Instruments – Category 1 (406736-2011) grants from the Natural Sciences and Engineering Research Council of Canada (NSERC) and the UQTR Research Chairs Program awarded to BB. The author would also like to thank Arnaud Côté-Boisvert and Isabelle Fafard for helping with data collection.
CONFLICTS OF INTEREST
The authors declare that they have no conflicts of interest.