INTRODUCTION
Chronic liver diseases (CLD) is the primary cause of morbidity rate and mortality around the globe,1 and the latest data from National Vital Statistics Reports showed that liver diseases are the 11th major cause of death in the United States. The causes of CLD include chronic viral and alcoholic hepatitis, cholestasis, and autoimmune and metabolic diseases, all of which may develop into liver cirrhosis and hepatocellular carcinoma. CLD patients often have non-specific symptoms, including fatigue, muscular and articular pains, inappetence, digestion problems, anxiety, depression, and other emotional problems, which degrade their quality of life and sense of happiness.1 In addition, CLD creates burdens on social resources and has a negative influence on individual patients and social welfare.2 From the angle of public health, it is important to know the causes and therapeutic methods for CLD. To prevent the occurrence of CLD and consider the patients’ life safety, the development of natural and safe liver protection supplements without side effects deserves in-depth research. Natural constituents are expected to improve hepatic metabolism, enhance the antioxidant capacity of the liver, and reduce liver fibrosis in patients with chronic hepatitis. CCl4 is extensively used in animal models for liver injury and liver fibrosis. Some studies have indicated that antioxidants prevent CCl4 hepatotoxicity by inhibiting oxidation and enhancing antioxidase activity.3
Natural products are the source of income when being used as promising drugs, and they deserve wider and deeper explorations. Many natural products manifest very strong antioxidant activity, and their bioactive constituents, such as polyphenols, flavonoids, and polysaccharides, have been proven able to provide different benefits to health, revealing their potential to be developed into effective therapeutic agents.4–6
As a part of the traditional Mediterranean diet, artichoke is eaten extensively. In preclinical and clinical research, artichoke extract has shown potential for lowering low-density lipoprotein (LDL) and as a liver protecting agent. Artichoke supplements can reduce alanine transaminase (ALT), aspartate transaminase (AST), total cholesterol, low-density lipoprotein-cholesterol (LDL-C), high-density lipoprotein cholesterol (HDL-C), and triglyceride concentrations in the serum. Studies have indicated that the supplementation of artichokes is beneficial to the livers of non-alcoholic fatty liver disease (NAFLD) patients.7 Artichoke supplements can inhibit the inflammation and cell apoptosis induced by H2O2 (the oxidative stress induced in HepG2 cells). Studies have indicated that in the NAFLD development process, artichoke supplements can inhibit the inflammation and apoptosis of liver cells directly and may help to prevent the progression of liver diseases, including hepatic steatosis and non-alcoholic steatohepatitis.8
Kenponashi have been used as a folk drug for centuries, especially in Japan, China, and Korea. Some parts of Japanese raisin tree reflect different health effects. The peduncle of Japanese raisin tree has antioxidant and immunostimulation effects, the fruit or stem shows antidiabetic effects through the adenosine monophosphate (AMP)-activated protein kinase pathway, and the root can inhibit the proliferation of hepatic stellate cells-T6 cells.9,10 Studies have indicated that the edible kenponashi can accelerate alcohol degradation and reduce alcohol concentrations in the blood.11 Kenponashi have strong antioxidant activity and contain remarkable phenols and flavonoids, as well as a small amount of polysaccharides. Kenponashi can alleviate oxidative liver injuries induced by alcohol, reduce the amount of alanine aminotransferase, aspartate aminotransferase, alkaline phosphatase, and triglyceride in the serum, increase the amount of glutathione (GSH) and superoxide dismutase (SOD) in the liver, increase the catalase activity, and reduce the amount of malondialdehyde and the amount of triglyceride in the liver. The analysis results of network pharmacology show that kaempferol, stigmasterol, and naringenin are the main bioactive constituents of Japanese raisin tree. The protection mechanism of against alcoholic liver disease (ALD) relates to regulating oxidation reactions, inflammation, enterogenous products, and cell apoptosis.12
During the past decade, increasing evidence has shown that sanghuangporus sanghuang can protect the liver from fibrosis due to its oxidation resistance. A study in 2002 showed that sanghuangporus sanghuang extract can restore catalase and SOD activity and restore the expression of aerobic respiration enzymes by reducing peroxidation products, so as to inhibit CCl4-induced late-stage liver fibrosis.13 It has been proved that sanghuangporus sanghuang polysaccharide can inhibit cytochrome P-450 (CYP) isoenzymes in the liver. Additionally, it has been reported that the retinoic acid derivative separated from sanghuangporus sanghuang reduces the early liver fibrosis induced by transforming growth factor-β through lowering the generation of active oxygen and inhibiting the expression of several proteins.13
The main active ingredient of fructus schisandrae is schisandrin B, which has multiple pharmacological activities, including antioxidant, anti-inflammation, anti-tumor, and liver protection.21 Some studies have indicated that schisandrin B protects liver cells from liver injuries induced by CCl4 and paracetamol by inhibiting the bioactivation induced by CYP and regulating the nuclear factor-erythroid 2-related factor 2 antioxidant response element (Nrf2-ARE) and antioxidant response element (ARE).17-19 Nuclear factor- erythroid 2-related factor 2 (Nrf2), a transcription factor that stimulates ARE, can protect multiple tissues and cells from oxidation reactions. Additionally, studies have indicated that schisandrin B inhibits the fibrosis signaling mediated by transforming growth factor-β (TGF-β) in A7r5 vascular smooth muscle cells and alpha mouse liver 12 (AML12) cells. All of the above findings showed that schisandrin B can protect liver cells from injury and inhibit the activation of hepatic stellate cells induced by TGF-β, indicating that it could be used to treat liver fibrosis.
The lignan in sesame has effective antioxidant and antiinflammatory properties. Sesamin is the most abundant lignan in sesame oil. Prior findings have shown that sesamin has antioxidant properties,20 anti-inflammatory action,21,22 antihypertensive effects,23 and cholesterol lowering effects24 as well as provides liver protection,25,26 neuroprotection,27 and cartilage protection.21 Liver inflammation is regarded as an index of liver fibrosis, which will develop into liver cirrhosis. The injury in relation to liver inflammation is caused by tumor necrosis factor-alpha (TNF-α), interleukin1b (IL-1b) and cyclooxygenase-2 (COX-2), which are pro-inflammatory cytokines. Sesamin weakens the strength of interleukin-6 (IL-6) and COX-2 in the liver induced by CCl4 by inhibiting the activation of nuclear factor-kB (NF-kB), so as to improve the pathological damage caused by liver fibrosis.3
Several vitamins, including A, C, E, and B12, have been recognized as antioxidants and have shown hepatoprotective effects against the hepatotoxicity caused by acetaminophen overdose.29 Vitamin B complex has a protective effect on fatty liver in rats, and its mechanism of action is to inhibit lipid metabolism disorder and resist oxidative damage, and can reduce serum and liver total cholesterol, triglyceride and ALT.30 In addition to increasing the activity and concentration of endogenous antioxidants in the liver, vitamin E can also reduce the concentration of lipid peroxidation in the liver.
The benefit of these constituents to the human body is known, but the use of this novel combination for improving hepatitis and liver fibrosis has not been studied or discussed. This experiment aimed to discuss artichoke compound (AHC) and used a CCl4-induced Wistar rat liver fibrosis model to evaluate the liver protection of AHC.
MATERIALS AND METHODS
Supplement Composition
The AHC was provided by HealthTake Corporation at a dosage of 768.7 mg per tablet. The formula contained artichoke extract, kenponashi extract, sanghuangporus sanghuang extract, fructus schisandrae extract, sesame extract, vitamin B complex, and vitamin E.
Dose Calculation
The recommended dosage of AHC for adults is four tablets a day (3,075 mg). The oral dose for the rats was calculated according to the metabolic conversion ratio of 6.2 between humans and rats, using the formula of 3,075 mg/60 kg (human body weight)×6.2=318 mg/kg. The doses used in this experiment were 318, 636, and 1,590 mg/kg per day, which were one, two, and five times the human dosage, respectively. The AHC was incorporated into 31.8, 63.6, and 159.0 mg/mL suspensions with 0.5% carboxylmethyl cellulose (CMC), and the rats were dosed at 1 mL/100 g body weight. The rats in the control group were given a CMC solution of the same volume.
Study Design
This study was approved by China Medical University’s Institutional Animal Care and Use Committee (No. of approval: CMUIACUC-2020-409) and performed according to China Medical University’s code of institutional animal ethics. A total of 50 sixweek-old male Wistar rats were purchased from BioLASCO Taiwan and raised in the animal room of China Medical University. The animal room temperature was set at 22±2 °C, and the illumination was set as 12-hours of light and 12-hours of darkness (light at 8 a.m. and dark at 8 p.m.). The animal experiment was performed after a one-week adaptation. The feed was imported from overseas (Prolab RMH 2500), and the drinking water was autoclaved. On the first day of administering the test materials, the rats were randomly divided into appropriate weight groups according to their body weights, with ten rats per group. The experimental animals were weighed once a week as the basis for administering the test materials. The CCl4 was dissolved in olive oil to prepare a 20% solution, and the dosage each time was 0.2 mL/100 g body weight.
The 50 rats were divided into the control group and the CCl4 experimental group. Then rats in the control group were given CMC once per day. The CCl4 experimental group was further divided into four groups, which were given CMC, low AHC (318mg/kg), medium AHC (636 mg/kg), and high AHC (1,590 mg/kg) doses once per day. The AHC was administered for one week before the CCl4 was administered. Afterwards, the control group was given olive oil in oral form twice per week (0.2 mL/100 g body weight) for eight weeks, and the CCl4 experimental group was given 20% CCl4 orally twice a week for eight-weeks. The CCl4 was administered between 9:00 a.m. and 9:40 a.m. each time. The AHC or CMC was administered between 11:00 a.m. and 11:40 a.m. once per day during the experiment (nine-weeks) except on days in which blood was sampled. The blood of all rats was sampled one week before the CCl4-induced liver injury and during weeks 0, 1, 3, and 6, and feeding was halted at 8 p.m. the night before blood sampling (light on at 8 a.m. and light off at 8 p.m. in the animal room of this university). The AHC or CMC was administered at 8 a.m. on the blood sampling day. Four hours after the AHC or CMC was administered, blood was drawn through the caudal artery of each narcotized rat to test the biochemical function of the rat’s livers. In week 8, blood was drawn through the abdominal aorta of each narcotized rat, and the rats were sacrificed at the same time. The liver and spleen of each rat were taken out quickly, cleaned with a cold physiological saline solution, dried and weighed (absolute weight). The relative weight (%) was worked out based on the corresponding body weight. The larger right lobe of a rat’s liver was divided into two parts, and the same regions were immersed in a 10% neutral formalin solution for the pathological section and then weighed and fully dried at 100 °C to determine the collagen content. The remaining liver parts were placed into four bags and stored at -80 °C for future use.
Biochemical Plasma Experiment
The obtained blood was centrifuged at 4,700 rpm for 15 minutes to obtain plasma for the biochemical experiments. The ALT and AST were investigated during weeks 1, 3, 6, and 8. Besides ALT and AST, the albumin, cholesterol, and triglycerides were investigated during week 8. The commercially available experiment reagents for albumin (MeDiPro Albumin Test (BC-005B), Formosa Biomedicaln Technology Corp., Taiwan), cholesterol (Cholesterol CHOP-PAP, Fortress diagnostics, UK), and triglycerides (Triglycerides FS, DiaSys Diagnostic Systems GmbH, Germany) were used, and a biochemical autoanalyzer (COBAS MIRA, Roche Diagnostics, Swiss) for serum was used for analysis.
Glutathione Assay for Liver Tissues
Glutathione in hepatic tissues was determined according to the method of Neuman et al,32 in which 0.5 g of liver tissue was weighed out and put in 5 ml of a 1.15% KCl solution before homogenization using a homogenizer. Next, 1 ml of homogenate was thoroughly mixed with 1 ml of trichloroacetic acid 10% and centrifuged at 3,000 g for 15 minutes. Then, 0.01 ml of the supernatant was put into 0.18 ml of a phosphate-EDTA buffer solution and a freshly prepared 0.01 ml σ-phthalaldehyde (1 mg/ml methanol) solution. After being mixed uniformly, the solution was detected by the fluorescence of 420 nm and excitation wavelength of 350 nm. The unit was represented by μmole per gram of tissue.
Determination of Protein Content in the Liver Tissue
The liver protein was determined using the supernatant of liver tissue homogenate of lipid peroxidation determination and was colored by a commercially available protein determination reagent (Coomassie Blue ( Kenlor Indus. Inc.; USA)). The absorbance value was measured at 540 nm. Bovine serum albumin was used as a standard and the protein content was represented by mg per gram of tissue.
Determination of Hydroxyproline Content in Liver Tissue
The hydroxyproline content was determined by referring to the method of Folch et al.33 The dry liver tissue was hydrolyzed and oxidized by H2
O2 and then colored by p-dimethylaminobenzaldehyde. The absorbance value was measured at 540 nm. The hydroxyproline content was represented by μg per gram of tissue.
Determination of Cholesterol and Triglyceride Concentrations in the Liver Tissue
The lipids were extracted according to the method of Folch et al33
and the triglyceride and cholesterol concentrations in the livers were measured.34 Liver tissue in the amount of 0.1 g was put into 2 mL of an extraction solvent (chloroform:methanol ratio=2:1) and homogenized by a homogenizer. The solution was then kept still at room temperature for 60-minutes before being centrifuged at 5,000 rpm for five minutes, after which the supernatant liquid was put into a clean 1.5 mL centrifuge tube and thoroughly mixed with 0.2 mL of 0.9% NaCl. The white and turbid liquid was centrifuged at 2,000 rpm for five minutes, and two layers were formed. The lower layer was placed in a dry heater for blow drying using nitrogen at 55 °C. Afterwards, it was mixed with 100 μL of solvent (tert-butyl alcohol: triton X-100: methanol=2:1:1),
heated at 65 °C for 15-minutes, and experimented upon using commercially-available cholesterol reagents (Cholesterol CHOPPAP, Fortress diagnostics, UK) and triglyceride reagents (Triglycerides FS, DiaSys Diagnostic Systems GmbH, Germany).
Determination of the SOD, Catalase, and Glutathione Peroxidase (GSH-Px) Activity in the Liver Tissue
Superoxide dismutase:
The tissues were pretreated according to the method of Aebi.35 The activity was determined by using commercially available SOD activity testing reagents (Ran-sod -RANDOX Lab. Ltd. UK). The SOD activity was defined as the enzyme content for inhibiting the 2-(-iodophenyl)-3-(4-nitrophenol)-5- phenyltetrazolium chloride reducing reaction rate by 50%,of which, this amount was defined as one unit (U) and represented by U per milligram of protein. Catalase: The catalase activity was determined according to the method of Sturgill et al. 36 The activity was defined as K (the rate constant of first-order reaction; min-1) as one unit (U), represented by U per milligram of protein. The computing method of K=(2.3/t2-t1) (logA1/A2), wherein A1 is the absorbance value when t1=0 seconds, and A2 is the absorbance value when t2=25seconds. Glutathione peroxidase: Liver tissues were pretreated according to the method of Aebi.35 The GSH-Px activity was determined using commercially available GSH-Px activity testing reagents (Ransel -RANDOX Lab. Ltd. UK)). The GSH-Px activity was defined as the enzyme content for oxidizing 1 μmol of nicotinamide adenine dinucleotide phosphate (NADPH) per minute, of which this content was defined as one unit (U). The GSH-Px activity of the liver tissues was represented by mU per milligram of protein. Pathological Examination The liver tissue was fixed by formalin, embedded in paraffin, and sliced. Two staining methods were used: a general Hematoxylin and Eosin stain (H.E. stain) and a Sirius Red stain (a special collagen stain). The liver injuries were divided into four classes and graded according to their pathology. Statistical Analysis The experimental data were analyzed by one-way analysis of variance, and Duncan’s multiple range test was performed. There was a significant difference between groups when p<0.05.
RESULTS
Influence on Plasma AST and ALT Activity As shown in Tables 1 and 2, the rats in the CCl4 +CMC group were dosed with CCl4, and the plasma AST and ALT values in weeks 1, 3, 6, and 8 were significantly higher than those of the control group. The rat plasma AST and ALT of the AHC-L plasma ALT and AHC-H groups in week 1 were significantly lower than those of the CCl4+CMC group. In week 3 and week 8, the rat plasma AST and ALT of the three dose groups of AHC were significantly lower than those of the CCl4+CMC group. The plasma ALT of the AHC-L and AHC-H groups was significantly lower than that of the CCl4+CMC group in week 6, and the plasma AST of the three dose groups of AHC was significantly lower than that of the CCl4+CMC group. As shown in Table 3, the rat plasma cholesterol and triglyceride concentrations of the CCl4+CMC group were significantly higher than those of the control group in week 8. The rat plasma triglyceride and cholesterol concentrations of the three dose groups of AHC were significantly lower than those of the CCl4+CMC group.
Table 1. Influence of AHC on the Plasma AST Value of Rats with CCl4 -induced Chronic Hepatitis |
Treatments
|
Week 1 (U/L)
|
Week 3 (U/L)
|
Week 6 (U/L)
|
Week 8 (U/L)
|
Control |
70.0±4.3a |
73.8±4.9a |
67.8±7.8a |
73.4±7.2a |
CCl4 +CMC |
169.7±36.5c |
584.4±171.2c |
1905.0±359.9c |
2340.5±346.6c |
CCl4 +AHC-L |
126.7±49.3bc |
360.3±147.1b |
1219.0±511.5b |
1797.1±604.0b |
CCl4 +AHC-M |
148.9±71.0bc |
426.3±162.6b |
1477.0±362.1b |
1814.0±492.1b |
CCl4 +AHC-H |
121.9±47.0b |
322.8±104.0b |
1275.0±713.5b |
1725.8±689.8b |
Control, administer once a day CMC; CCl4 +CMC, 20% CCl4 +CMC; CCl4 +AHC-L, 20% CCl4 +318 mg/ kg AHC; CCl4 +AHC-M, 20% CCl4 +636 mg/kg AHC; CCl4 +AHC-H, 20% CCl4 +1590 mg/kg AHC. Values were expressed as means±SD. Each value is a mean of 10 observations. Value bearing different superscrits within a colum differ significantly at 5% level (p<0.05) |
Influence on Liver and Spleen Weight
As shown in Table 4, after the rats in the CCl4+CMC group were dosed with CCl4, the final absolute and relative weights of the spleen were significantly higher than those of the control group, and the absolute and relative weights of the spleen for the three dose groups of AHC were significantly lower than those of the CCl4+CMC group. As shown in Table 4, the rats in the CCl4+CMC group were dosed with CCl4, and the final absolute weight and relative weight of their livers were significantly higher than those of the control group. The absolute weight and relative weight of the livers of the three dose groups given AHC did not differ from those of the CCl4+CMC group.
Table 2. Influence of AHC on the Plasma ALT Value of Rats with CCl4 -induced Chronic Hepatitis |
Treatments
|
Week 1 (U/L)
|
Week 3 (U/L)
|
Week 6 (U/L)
|
Week 8 (U/L)
|
Control |
41.0±4.0a |
45.1±4.8a |
44.4±6.1a |
42.1±4.4a |
CCl4 +CMC |
144.2±30.3c |
494.4±146.4c |
1675.0±406.4c |
1959.1±388.4c |
CCl4 +AHC-L |
93.7±34.0b |
220.5±104.1b |
1270.0±355.1b |
1348.6±707.9b |
CCl4 +AHC-M |
115.0±70.6bc |
294.9±140.5b |
1341.0±360.9bc |
1465.2±409.8b |
CCl4 +AHC-H |
88.7±34.0b |
217.8±68.1b |
1250.0±567.5b |
1381.7±537.3b |
Control, administer once a day CMC; CCl4 +CMC,20% CCl4 +CMC; CCl4 +AHC-L, 20% CCl4 +318 mg/ kg AHC; CCl4 +AHC-M, 20% CCl4 +636 mg/kg AHC; CCl4 +AHC-H, 20% CCl4 +1590 mg/kg AHC. Values were expressed as means±SD. Each value is a mean of 10 observations. Value bearing different superscrits within a colum differ significantly at 5% level (p<0.05). |
Table 3. Influence of AHC on the Plasma Albumin, Cholesterol and Triglyceride Concentrations of Rats with CCl4 -induced Chronic Hepatitis |
Treatments
|
Albumin (g/dL)
|
Cholesterol (mg/dL)
|
Triglycerides (mg/dL)
|
Control |
3.41±0.20c |
71.1±13.2a |
67.2±19.9a |
CCl4 +CMC |
2.62±0.41a |
125.4±50.1b |
164.0±55.7c |
CCl4 +AHC-L |
3.04±0.26b |
90.6±34.9a |
96.9±30.8ab |
CCl4 +AHC-M |
2.90±0.24b |
94.1±28.0a |
122.1±38.4b |
CCl4 +AHC-H |
3.09±0.12b |
83.8±35.4a |
97.0±49.6ab |
Control, administer once a day CMC; CCl4 +CMC,20% CCl4 +CMC; CCl4 +AHC-L, 20% CCl4 +318 mg/kg AHC; CCl4 +AHC-M, 20% CCl4 +636 mg/kg AHC; CCl4 +AHC-H, 20% CCl4 +1590 mg/kg AHC. Values were expressed as means±SD. Each value is a mean of 10 observations. Value bearing different superscrits within a colum differ significantly at 5% level (p<0.05). |
Influence on Liver Protein, GSH, Hydroxyproline, Cholesterol, and Triglyceride Concentrations
As shown in Table 5, the CCl4-induced chronic hepatitis in the rats caused the soluble protein concentration in their livers to be significantly lower than that of the control group, while the liver protein concentration of the three dose groups of AHC did not differ from that of the CCl4+CMC group. As shown in Table 5, the CCl4-induced chronic hepatitis in the rats caused the liver GSH concentration to be significantly lower than that of the control group, while the liver GSH concentration in the AHC-L and AHC-H groups was significantly higher than that of the CCl4+CMC group. The AHC-M group showed an uptrend, but there was no statistical significance. As shown in Table 5, the CCl4-induced chronic hepatitis in the rats obviously increased the liver hydroxyproline concentration. The liver hydroxyproline concentration of the three dose groups of AHC was apparently lower than that of the CCl4+CMC group. The CCl4- induced chronic hepatitis in the rats caused the liver cholesterol concentration to be significantly higher than that of the control group, while the liver cholesterol concentration in the AHC-H group was significantly lower than that of the CCl4+CMC group. The CCl4-induced chronic hepatitis in the rats caused the liver triglyceride concentration to be significantly higher than that of the control group, while the liver triglyceride concentration in the three dose groups of AHC did not differ from that of the CCl4 +CMC group.
Table 4. Influence of AHC on the Absolute and Relative Weights of the Spleen and Liver of Rats with CCl4 -induced Chronic Hepatitis |
Treatments
|
Spleen (g)
|
Spleen (%)
|
Liver (g)
|
Liver (%)
|
Control |
1.09±0.09a |
0.22±0.02a |
14.0±1.6a |
2.9±0.2a |
CCl4 +CMC |
1.98±0.54c |
0.46±0.14c |
17.4±5.5b |
4.0±1.2b |
CCl4 +AHC-L |
1.32±0.37ab |
0.31±0.11ab |
18.0±2.6b |
4.1±0.9b |
CCl4 +AHC-M |
1.55±0.31b |
0.37±0.11b |
19.9±3.5b |
4.7±1.2b |
CCl4 +AHC-H |
1.35±0.22ab |
0.31±0.06ab |
18.4±3.0b |
4.1±0.7b |
Control, administer once a day CMC; CCl4 +CMC, 20% CCl4 +CMC; CCl4 +AHC-L, 20% CCl4 +318 mg/ kg AHC; CCl4 +AHC-M, 20% CCl4 +636 mg/kg AHC; CCl4 +AHC-H, 20% CCl4 +1590 mg/kg AHC. Values were expressed as means±SD. Each value is a mean of 10 observations. Value bearing different superscrits within a colum differ significantly at 5% level (p<0.05). |
Table 5. Influence of AHC on the Liver Protein, GSH, Hydroxyproline, Cholesterol and Triglyceride Concentrations of Rats with CCl4 -induced Chronic Hepatitis |
Treatments
|
Protein (mg/g tissue)
|
GSH (μmol/g tissue)
|
Hydroxyproline (μg/g tissue)
|
Cholesterol (mg/g tissue)
|
Triglycerides (mg/g tissue) |
Control |
115.6±25.7b |
5.7±1.0c |
205.7±55.2a |
3.1±0.4a |
40.1±8.3a |
CCl4 +CMC |
85.3±23.7a |
3.4±1.3a |
10007.9±332.8c |
4.7±0.8c |
74.9±14.6b |
CCl4 +AHC-L |
103.5±27.4ab |
5.3±1.9bc |
611.1±252.3b |
4.3±0.8bc |
68.1±17.7b |
CCl4 +AHC-M |
89.5±23.4a |
4.2±1.5ab |
778.5±279.3b |
4.7±0.9c |
79.9±12.1b |
CCl4 +AHC-H |
108.4±19.2ab |
5.5±0.9bc |
604.7±178.6b |
3.8±0.4b |
71.4±18.2b |
Control, administer once a day CMC; CCl4 +CMC, 20% CCl4 +CMC; CCl4 +AHC-L, 20% CCl4 +318 mg/ kg AHC; CCl4 +AHC-M, 20% CCl4 +636 mg/kg AHC; CCl4 +AHC-H, 20% CCl4 +1590 mg/kg AHC. Values were expressed as means±SD. Each value is a mean of 10 observations. Value bearing different superscrits within a colum differ significantly at 5% level (p<0.05). |
Influence on Liver SOD, Catalase and GSH-Px Activities
As shown in Table 6, the CCl4-induced chronic hepatitis in the rats caused the activities of antioxidant enzymes of SOD, catalase, and GSH-Px in the liver to be apparently lower than that of the control group. The liver SOD activity of the three dose groups of AHC did not differ from that of the CCl4+CMC group. The liver GSH-Px and catalase activities of the AHC-H group were significantly higher than that of the CCl4+CMC group. The liver GSHPx and catalase activities of the AHC-L and AHC-M groups did not differ from those of the CCl4+CMC group.
Table 6. Influence of AHC on the Liver SOD, Catalase and GSH-Px Activities of Rats with CCl4 -induced Chronic Hepatitis |
Treatments
|
SOD
(U/mg protein) |
Catalase
(U/mg protein) |
GSH-Px
(mU/mg protein)
|
Control
|
16.6±2.9b |
13.0±2.6b |
1831.6±412.2c
|
CCl4 +CMC
|
10.6±5.0a |
7.8±1.9 |
915.4±327.3a
|
CCl4 +AHC-L
|
12.1±5.3ab |
10.5±3.9ab |
1228.6±519.7ab
|
CCl4 +AHC-M
|
12.1±5.4a |
9.3±4.7ab |
1160.3±341.8ab
|
CCl4 +AHC-H
|
12.1±5.5ab |
12.1±5.0b |
1441.7±597.4bc
|
Control, administer once a day CMC; CCl4 +CMC,20% CCl4 +CMC; CCl4 +AHC-L, 20% CCl4 +318 mg/kg AHC; CCl4 +AHC-M, 20% CCl4 +636 mg/kg AHC; CCl4 +AHC-H, 20% CCl4 +1590 mg/kg AHC. Values were expressed as means±SD. Each value is a mean of 10 observations. Value bearing different superscrits within a colum differ significantly at 5% level (p<0.05). |
Pathological Changes
As shown in Table 7 and Figure 1, the CCl4-induced chronic hepatitis in the rats stained by H.E. showed apparent tissue vacuolization and necrosis (Figure 1 B). The liver tissue vacuolization and necrosis degrees of the three dose groups of AHC did not differ from those of the CCl4
+CMC group.
Figure 1. Rat Liver Tissue Structure (H.E. staining)
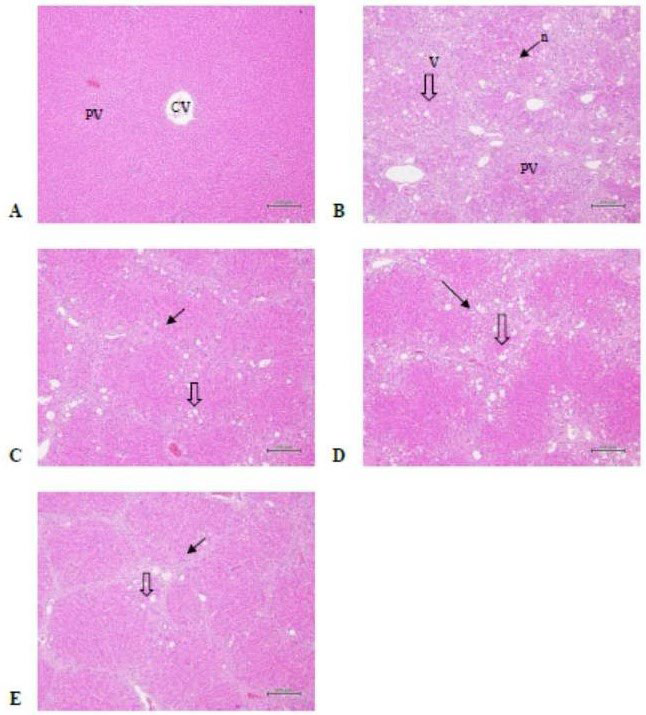
A) Normal liver tissue structure of the normal control group (animal No.: NV1-4);
B) Liver vacuolization and necrosis scores is 2.9±0.9 and 1.5±0.7 of the CCl4 group (animal No. :CMC4-2)
C) Liver fibrosis score is 2.8±0.7 and 1.4±0.5 of the low dose group (animal No. :L3-3);
D) Liver fibrosis score is 3.5±0.5 and 1.1±0.3 of the medium dose group(animal No. :M3-2);
E) Liver fibrosis score is 2.9±0.8 and 1.4±0.5 of the high dose group (animal No. :H1-1) v: vacuolization; n: necrosis; CV: central veins; PV: portal trigone
As shown in Table 7 and Figure 2, tissue fibrosis could be observed clearly after Sirius Red staining (Figure 2B). The degree of liver tissue fibrosis in the three dose groups of AHC was significantly lower than that of the CCl4+CMC group.
Figure 2. Rat Liver Tissue Structure (Sirius Red staining)
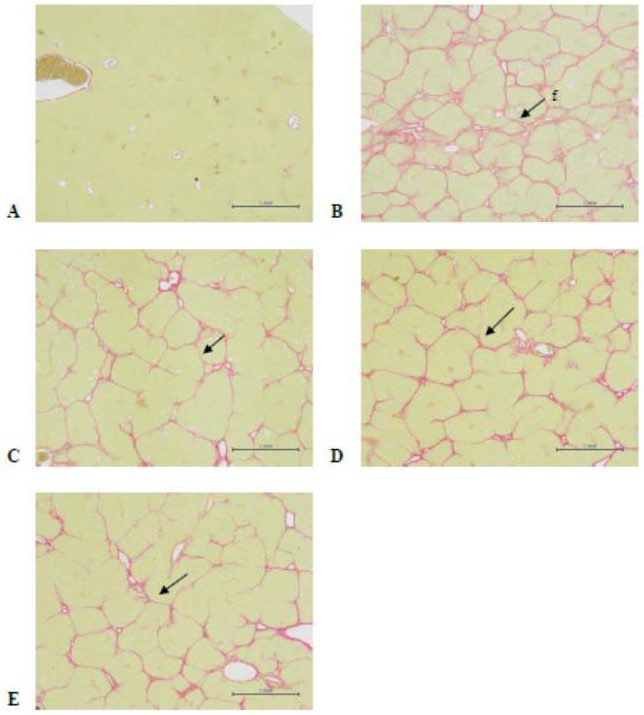
A) Normal liver tissue structure of the normal control group (animal No.: NV1-4);
B) Liver fibrosis score is 3.7±0.5 of the CCl4 group (animal No. :CMC4-2) ;
C) Liver fibrosis score is 2.6±0.7 of the low dose group (animal No. :L3-3) ;
D) Liver fibrosis score is 3.1±0.7 of the medium dose group(animal No. :M3-1) ;
E) Liver fibrosis score is 2.7±0.8 of the high dose group (animal No. :H1-1) 40×’Bar=1 mm; f:fibrosis; CV: central veins; PV: portal trigone
Table 7. Influence of AHC on the Liver Histology of Rats with CCl4 -induced Chronic Hepatitis |
Treatments
|
Vacuolization
(score) |
Necrosis
(score) |
Fibrosis
(score) |
Control
|
0.0±0.0a |
0.0±0.0a |
0.0±0.0a
|
CCl4 +CMC
|
2.9±0.9b |
1.5±0.7b |
3.7±0.5c
|
CCl4 +AHC-L
|
2.8±0.7b |
1.4±0.5b |
2.6±0.7b
|
CCl4 +AHC-M |
3.5±0.5b |
1.1±0.3b |
3.1±0.7b |
CCl4 +AHC-H
|
2.9±0.8b |
1.4±0.5b |
2.7±0.8b
|
Control, administer once a day CMC; CCl4 +CMC,20% CCl4 +CMC; CCl4 +AHC-L, 20% CCl4 +318 mg/kg AHC; CCl4 +AHC-M, 20% CCl4 +636 mg/kg AHC; CCl4 +AHC-H, 20% CCl4 +1590 mg/kg AHC. Values were expressed as means±SD. Each value is a mean of 10 observations. Value bearing different superscrits within a colum differ significantly at 5% level (p<0.05). |
When the liver is damaged, AST and ALT leak out of the liver cells and cause plasma AST and ALT activities to increase, and they are the most frequently used biochemical indexes of liver injury.37 ALT is relatively specific, whereas AST exists in the heart, kidneys, skeletal muscles, and brain.37 Liver injury was induced by CCl4 in this experiment, and the activities of AST and ALT in the plasma increased obviously. AHC can reduce the AST and ALT activities in the serum, meaning that it can alleviate the damage of CCl4 to the liver. The albumin present in plasma
mainly comes from hepatic synthesis, and the plasma albumin content decreases when chronic hepatitis induces hepatic fibrosis.38 Due to the CCl4 -induced chronic hepatitis in the rats in this experiment, the plasma albumin decreased in week 8, and the final soluble protein content in the liver tissue decreased obviously. The AHC could increase the albumin in the plasma and the soluble protein content in the liver tissue, meaning it could improve the liver protein synthesis hypofunction induced by CCl4.
Blood flow encounters resistance when entering a liver affected by hepatic fibrosis, and this can lead to portal hypertension. Subsequently, the splenic blood flow is influenced, and the spleen swells.39 In this experiment, the CCl4-induced chronic hepatitis eventually led to spleenomegaly. The AHC caused a reduction in spleen size, meaning it could mitigate portal hypertension. Chronic hepatitis can induce hepatic fibrosis, i.e., connective tissue proliferation. Connective tissue is mainly composed of collagen. As hydroxyproline is a specific component of collagen, the collagen content can be reflected by the hydroxyproline content to represent the degree of fibrosis.40 In terms of the CCl4-induced chronic hepatitis in this experiment, the liver hydroxyproline content showed an obvious increase. The AHC reduced the hydroxyproline content, meaning it could alleviate liver fibrosis. This effect was further verified by a pathological examination of the tissues.
GSH participates in numerous liver cell functions, such as detoxification, scavenging free radicals, and regulating the cell cycle.41,42 In this experiment, the CCl4 treatment reduced the liver GSH content, and the AHC treatment increased the liver GSH content. CCl4 induced chronic liver injury in this experiment and caused the activities of the free radical scavenging enzymes of SOD, catalase, and GSH-Px in the liver to decline obviously. The AHC did not improve the SOD activity, but a high-dose of AHC enhanced the GSH-Px and catalase activities. The results show that AHC could alleviate the harm caused by free radicals by increasing the antioxidant, GSH, content in the liver.
In this experiment, the AHC contained artichoke extract. Current research has shown that in non-alcoholic steatohepatitis (NASH), the active ingredients of herbs, such as flavonoids and caffeoylquinic acid, have been proven to have liver protection activities.43 Flavonoids are effective antioxidants and free radical scavengers that can prevent liver injury. The liver protection of kenponashi extract has been mentioned in multiple pieces of literature,42,44 showing its protective abilities toward the liver. The protective effects of kenponashi extract on acute alcohol-induced liver injury were investigated in vivo using mice as test models. In the present study, kenponashi extract (150, 300, 600 mg/kg/day) was given to mice by intragastric administration for 4-days. In mice, administration of kenponashi extract significantly decreased the activities of ALT and AST in serum. Administration of kenponashi extract also protected against alcohol-induced alcohol dehydrogenase elevation in mice. Concurrently, there was an augmentation in
the activities of antioxidant enzymes such as SOD, GST, and GSH,
and it also facilitated alcohol metabolism.42
One study found that sanghuangporus sanghuang polysaccharide extract can protect the liver from oxidative stress, especially by scavenging iron-related free radicals and regulating the metabolism of amino acids and nucleic acids, thereby improving liver operations. Sanghuangporus sanghuang polysaccharide extract can protect the rats’ livers from hepatic fibrosis via the aforementioned two ways.45 Overdose of acetaminophen is currently one of the main causes of hepatoxicity and acute liver injury, which is often linked to oxidative stress. Sanghuangporus sanghuang polysaccharide have shown many hepatoprotective effects. The results indicated that sanghuangporus sanghuang treatment effectively alleviated acetaminophen-induced acute liver injury by reducing ALT and AST levels in serum.46
Fructus schisandrae is a traditional liver protector, and some studies have shown that it has significant antioxidant activity and obvious protection against liver injuries induced by CCl4, alcohol, or a high-fat diet.47-49 Fructus schisandrae polysaccharide significantly reduced the liver index by 12.0%. Serum levels of triglycerides, total cholesterol, low-density lipoprotein cholesterol, alanine aminotransferase and aspartate aminotransferase were decreased by 31.3, 28.3, 42.8, 20.1 and 15.5%, respectively. Serum high-density lipoprotein cholesterol was increased by 26.9%. Further, fructus schisandrae polysaccharide lowered hepatic triglycerides and total cholesterol content by 27.0% and 28.3%, respectively, and alleviated fatty degeneration and necrosis of liver cells.48
Sesame extract can significantly reduce liver lipid accumulation. Furthermore, oxidative stress in the liver can be improved by increasing the GSH, vitamin C, and Nrf2 levels as well as reducing the malondialdehyde (MDA) and NO (nitric oxide) levels while enhancing the SOD, catalase, and GSH-Px activities.50 And sesamin was administered in two different dose (5 and 10 ml/kg bw) to evaluate the hepatoprotective activity. Sesamin significantly reduced the elevated serum liver marker enzymes (p<0.0001). Reduction of thiobarbituric acid reactive substances (TBARS) (p<0.01 and p<0.001) followed by enhancement of GSH., SOD and catalase (p<0.0001) in liver homogenate in sesamin treated groups shows the amelioration of oxidative stress induced by CCl4. Histopathological report also supported the hepatoprotection offered by sesaminFrom these above findings it has been concluded that sesamin ameliorate the oxidative liver injury in terms of reduction of lipid peroxidation and enhancement of liver antioxidant enzymes.51 The above findings all showed that sesame extract could protect against metabolic diseases in relation to NAFLD and could be used as an effective dietary supplement for improving liver diseases.
LIMITATION
Presently, preliminary significant results have been found in animal experiments. Whether long-term administration to the human body can improve the problems of chronic liver injuries is worthy of in-depth discussion and should be observed in the future.
CONCLUSION
The animal experiment results of this study showed that AHC had a number of effects on rat livers damaged by CCl4, including reducing the plasma ALT and AST values, increasing the plasma albumin concentration, alleviating splenomegalia, reducing the degree of hepatic fibrosis, and increasing the antioxidant, GSH content in the liver.
It was confirmed that for CCl4-induced rat liver injuries, AHC contributed to reducing the plasma ALT and AST values, increasing the antioxidant, GSH, content in the liver, and lowering the degree of hepatic fibrosis, so as to protect the liver.
ACKNOWLEDGEMENTS
This study was sponsored by HealthTake corporation, who provided the AHC used in this study.
CONFLICTS OF INTEREST
The authors declare that they have no conflicts of interest.