INTRODUCTION
Compression garments (CG) are commercially available and highly popular sports apparel amongst athletes and fitness enthusiasts. CGs were initially used to promote post-operative recovery for lymphedema1 and vascular disorders2 in clinical settings. Pressure applied by the CGs on the muscles, bones and connective tissue had an effect of limiting the area of swelling, aiding venous return and preventing blood from being pooled in the veins. Over years, CGs have made inroads into sports and athletic training and performance-related applications. It has subsequently grown in popularity that is reflected by the global revenue figures being forecasted to increase from approximately USD 23.87 billion in 2016 to USD 32.17 billion by the end of 2022.3 CGs have been proposed to assist in accelerating muscle recovery, reducing muscle soreness4 and improving athletic performance.5 These conclusions have stemmed from evidence suggesting the efficacy of CGs in minimizing muscle oscillations6,7 during exercise and reducing the extent of exercise-induced muscle damage (EIMD).8 There are many types of CGs available including compression tops such as sleeves and upper-body CGs that cover the limbs fully, partially or not at all, and compression bottoms such as shorts, long pants and calves. Different brands of CGs are made from various materials and offer the differing amount of compression. Competitive athletes wear CGs with the goal of improving their performance during and recovery after their events, even though the effects of CGs are not yet fully understood.
Current literature indicates that CG has the potential to improve muscle strength by reducing the extent of EIMD.9 Minimizing structural damage to muscle fibers and sarcomeres increases the contractile functions in skeletal muscles,10,11 enhancing the muscles’ ability to generate a ‘power stroke’ for muscle contraction and thus, increasing the maximum force output generated by muscles.12 As muscle strength is a determinant for running economy and is directly related to exercise tolerance, minimizing muscle strength loss using CGs could potentially lead to a faster rate of recovery and enhance athletic performance.13
In addition to the above effects, existing studies propose that CGs could reduce delayed onset muscle soreness (DOMS) by increasing blood circulation to fatigued muscles.14 Faster blood flow and enhanced venous return accelerates removal of metabolic by-products that contribute to muscle fatigue and soreness from exercising muscles, maintains force output,15 and increases time to exhaustion.16,17 A study on competitive cyclists reported improvement in hemodynamics, peak power and cycling performance in CG trials,18 suggesting that CG increased blood supply to the muscles, and facilitated the removal of metabolites associated with muscle fatigue and soreness.
While the body of evidence progressively grows with regard to the effects of CGs on athletic performance and recovery, a substantial part of the literature remains equivocal. Many studies have used subjective measures based on individual perceptions and provided theoretical speculations to report the effects of CGs on skeletal muscle physiology. Furthermore, there are conflicting studies reporting no effects of CGs on the recovery of muscle function or on EIMD.19,20 Despite the research on CGs over a decade, there are apparently few systematic reviews done in this area. To the best of our knowledge, according to the definition of systematic review by Centre for Cognitive Ageing and Cognitive Epidemiology (CCACE) and PRISMA-P terminology,21 there has been only one systematic review on the evidence related to effects of CGs on performance and recovery.7 A few other reviews14,22 that claimed to be systematic review papers were, in fact, a combination of systematic review and meta-analysis, as statistical analysis was included to review the papers that met their eligibility criteria. Therefore, the aim of this systematic review was to identify and evaluate the current literature on the effects of CGs on skeletal muscle physiology, performance, and recovery in young healthy adults. This systematic review, modeled after the PRISMA-P checklist,21 sought to consolidate the literature in the past decade to determine whether CGs provide any real performance and recovery-related benefits in healthy young adults.
METHODS
Eligibility Criteria
This systematic review examined all original published studies over the last decade (from Feb 2008 to Feb 2018) that used randomized controlled trials (RCTs), randomized repeated measures (RRMs) and randomized counter-balanced measures (RCMs) study designs. The population included were all young healthy adult males and females (18 to 30 years old) without any past or current injuries that might affect the results of the eligible studies. Studies that used a commercially available brand of sports CGs limited to sleeves, upper-body compression top fully, partially or not covering the limbs, shorts, pants, and calves were included. Comparison to a control group was also a necessary criterion. Eligible studies included only those published in English and reported results were related to skeletal muscle physiology, performance and recovery including but not limited to muscle oscillation, muscle oxygenation, force production, lactate clearance, and muscle enzyme (creatine kinase (CK) and lactate dehydrogenase (LDH)) clearance.
Data Sources and Literature Search
Eligible studies were sourced from electronic databases PubMed, SPORTDiscus and Web of Science. Keywords used included: “compression garments”, “compression clothing”, “compression stocking”, “young adults”, “healthy adults”, “exercise”, “physiology”, “performance”, “recovery”, “skeletal muscle physiology”, “skeletal muscle performance”, “fatigue”, “muscle damage” and “skeletal muscle recovery”. Additionally, the reference lists of these identified articles were manually searched for studies that matched the eligibility criteria.
Study Selection and Data Extraction
Studies from the databases were screened systematically and eliminated according to the inclusion and exclusion criteria during the different stages (Figure 1). Data from all selected studies were extracted using the data extraction form.
Figure 1: Study Identification, Screening and Exclusion Pathway
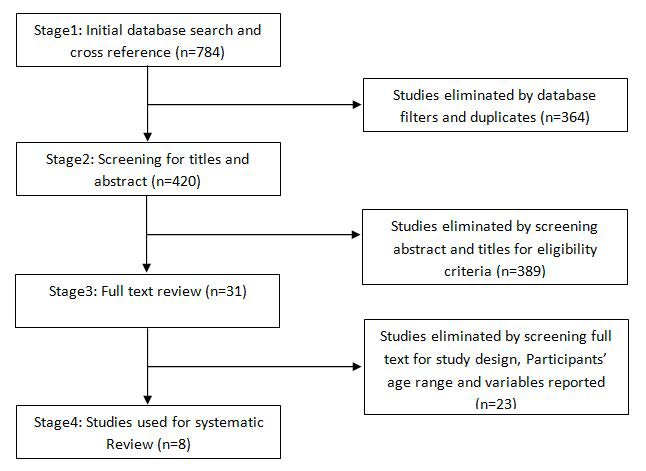
Risk of Bias in Individual Studies
Each selected eligible study was also evaluated by the Physiotherapy Evidence Database (PEDro) Scale.23 If the statements matched the evaluated study, a “yes’’ answer would add 1 point, and if it did not, a “no’’ would add 0 points. Since the first statement was excluded in the tabulation of the final score, the maximal score a study could get was 10 points. The PEDro Scale had been applied by past studies on CGs14,22 to access the methodological quality of their systematic review.
RESULTS
A total of 784 published papers were identified on initial database and cross-referencing search (Figure 1), of which eventually a fulltext systematic review was conducted for 31 studies. Finally, eight studies met the eligibility criteria and were used for systematic review.
Characteristics of the Eligible Studies
The eight eligible studies used for this systematic review included six RRMs and two RCMs. A total of 108 subjects were included, with 106 males (three studies didn’t specify subjects’ gender but assumed to be males) and 2 females, with fitness levels ranging from experienced gym users who performed strenuous resistance exercises to professional athletes who competed at national and international events (Table 1). All participants were between 18 to 30-years of age and did not have any injuries in the past 6-months that might affect the accuracy or validity of the studies.
Table 1. Characteristics and Summary Findings of the Eligible Studies |
Study |
Study design |
No. of Participants, gender, fitness level |
CG type |
Applied Pressure (mmHg) |
Exercise or recovery protocol |
Effects of CG on skeletal muscle |
Dascombe et al24 |
RRM |
7, 5 M 2 F, national flatwater kayakers |
UBCG with long sleeves |
Not reported |
six-step incremental test 4minPT |
Power ↔, Blood lactate ↔ , [O2Hb] ↔ , [tHb] ↔ Peak power ↔ , Mean power ↔ , [O2Hb] ↔ , [tHb] ↔ , Total work ↔ , Relative work ↔ |
Duffield et al19 |
RRM |
14, unknown, club-standard rugby players |
LBCG |
Not reported |
STG and HIT |
Peak power ↔, Blood lactate ↔, CK ↔ |
Duffield et al25 |
RCM |
11, unknown, Competitive rugby players playing at club- and regional- standard |
LBCG |
Not reported |
10 x 20m sprint and 100 SSC bounds |
Knee extensor peak twitch force ↔, Blood lactate ↔, CK ↔, C-RP ↔, AST ↔ |
Goto et al9 |
RRM |
9, 9 M, more than 2 years of experience with resistance training |
Full body CG |
Not reported |
10 x 9 strenuous resistance exercises |
Chest press 1RM ↑, knee extension 1RM ↑, Blood lactate ↔, Upper arm circumference ↓, thigh circumference ↓ |
Hamlin et al26 |
RRM |
22, 22 M, rugby union players |
CG pants |
~8 sphyrion, ~13 mid-calf, ~9 midtrochanterion |
10 x 40m sprints and 3km run |
CK ↔, blood lactate ↔ |
Lovell et al27 |
RCM |
25, unknown, semiprofessional rugby league players |
CG pants |
~20 ankle, ~15 calf |
6-stage submaximal treadmill test |
Blood lactate ↓ |
Pruscino et al28 |
RRM |
8, 8 M, highly trained national hockey players |
CG pants |
~5 thigh, ~7 calf, ~19 ankle |
LIST protoco |
Blood lactate ↔ , CK ↔ , SJ peak force ↔ , 5CMJ peak force ↔ , 5CMJ peak power ↓ |
Scanlan et al29 |
RRM |
12, 12 M, competitive cyclists |
CG pants |
~9 butt, ~15 hamstring, ~17 calf, ~20 ankle |
Incremental test 1h time trial |
Peak blood lactate ↔ , Peak [O2 Hb] ↔ blood lactate ↔, [O2 Hb] ↔ |
*no significant effect reported during other measurement times, except for 24h after wearing CG pants for recovery. [O2 Hb]=tissue oxyhaemoglobin; [tHb]=total haemoglobin; ↔=no significant effect compared to control group; ↑=increased significantly compared to control group; ↓=decreased significantly compared to control group; ~=approximately; 1RM=one repetition maximum; 4minPT=four-minute maximal performance test; AST=aspartate transaminase; CG=compression garment; CK=Creatine Kinase; CRP=c-reactive protein; F=female; HIT=high-intensity tests; LBCG=lower body compression garments; M=male; RCM=randomised counter-balanced measure; RRM=randomised repeated measure; STG=simulated team game; UBCG=upper body compression garments; 5CMJ=five repetition counter movement jumps; LIST=Loughborough Intermittent Shuttle Test; ; SJ=squat jump |
Six of the eight studies used lower body CG, while one study each used upper body CG24 and full body CG9 respectively. Only four studies that used lower body CGs reported the applied pressure of the garment, ranging from 5 mmHg to 20 mmHg. Three studies focused on skeletal muscle recovery, two studies on skeletal muscle performance and physiology,24,29 while three studies focused on skeletal muscle performance and recovery.
All studies reported blood lactate concentrations between CG group and control group during the performance and/or recovery. Other skeletal muscle variables reported across the eight studies included the concentration of CK, C-reactive protein (C-RP) and aspartate transaminase (AST), muscle oxygenation, peak and mean power, total and relative work, peak force and upper arm and thigh circumference.
Risk of Bias Across Studies
The risk of biases for each of the eight studies was evaluated by the physiotherapy Evidence Database (PEDro) Scale.23 The studies had an average score of 6.2 on the PEDro scale (Table 2), with the range from 6-7 points. All eight studies were deemed to have unclear risk of bias with regard to blinding of the therapists and assessors. Six studies except Hamlin et al26 and Pruscino et al28 were evaluated to have unclear risk of bias regarding blinding of subjects. However, due to the nature of the RCM or RRM study designs adopted by all eight studies, non-blinding of subjects, therapists and assessors were deemed not to have significant impacts on the reported results of these studies.
Table 2. Quality Assessment of the Eligible Studies Based on the PEDro Scale23 |
|
Study-1 (24)
|
Study-2 (19) |
Study-3 (25) |
Study-4 (9) |
Study-5 (26) |
Study-6 (27) |
Study-7 (28) |
Study-8 (29) |
Criteria met, n (%) |
Eligibility criteria were specified |
Yes |
Yes |
Yes |
Yes |
Yes |
Yes |
Yes |
Yes |
8 (100%)
|
Subjects were randomly allocated to groups (in a crossover study, subjects were randomly allocated an order in which treatments were received)
|
1 |
1 |
1 |
1 |
1 |
1 |
1 |
1 |
8 (100%) |
Allocation was concealed |
0 |
0 |
0 |
0 |
0 |
0 |
0 |
0 |
0 (0%)
|
The groups were similar at baseline regarding the most important prognostic indicators
|
1 |
1 |
1 |
1 |
1 |
1 |
1 |
1 |
8(100% |
There was blinding of all subjects |
0 |
0 |
0 |
0 |
0 |
0 |
0 |
0 |
2 (25%)
|
There was blinding of all therapists who administered the therapy
|
0 |
0 |
0 |
0 |
0 |
0 |
0 |
0 |
0 (0%) |
There was blinding of all assessors who measured at least one key outcome |
0 |
0 |
0 |
0 |
0 |
0 |
0 |
0 |
0 (0%)
|
Measures of at least one key outcome were obtained from more than 85% of the subjects initially allocated to groups
|
1 |
1 |
1 |
1 |
1 |
1 |
1 |
1 |
8 (100%) |
All subjects for whom outcome measures were available received the treatment or control condition as allocated or, where this was not the case, data for at least one key outcome was analysed by “intention-to-treat” |
1 |
1 |
1 |
1 |
1 |
1 |
1 |
1 |
8 (100%)
|
The results of between-group statistical comparisons are reported for at least one key outcome
|
1 |
1 |
1 |
1 |
1 |
1 |
1 |
1 |
8 (100%) |
The study provides both point measures and measures of variability for at least one key outcome |
1 |
1 |
1 |
1 |
1 |
1 |
1 |
1 |
8 (100%)
|
Total PEDro score
|
6/10 |
6/10 |
6/10 |
6/10 |
7/10 |
6/10 |
7/10 |
6/10 |
|
DISCUSSION
The strength of this review was in following the CCACE definition and PRISMA-P terminology of systematic review, including studies with robust methodological designs like RRM and RCM, a clear and consistent set of eligibility criteria, modelling of the review based on the PRISMA-P checklist, and using the PEDro scale to evaluate and eliminate the risk of bias in the selected studies. Therefore, although the total number of participants from the eight studies were 108, the methodological approach to these studies, and the minimised risk of bias provides a reasonably strong evidence on the possible ‘cause-and-effect’ relationship, and provides adequate bases to elucidate the mechanistic aspects of the effects of CGs on skeletal muscle physiology, performance and recovery measures in young healthy adults.
Summary of Evidence
Skeletal muscle physiology and performance: Studies in the past have focused on the effects of CGs on muscle physiology and performance during different types of exercise. Significant physiological effects have been reported including reduced muscle oscillation and improved proprioception,30 and improved muscle oxygenation.31 However, these studies did not include a control group, nor was the methodological design randomized. Therefore, the findings of the cited studies are likely to be of questionable validity. This systematic review included studies with more robust eligibility criteria and research design. Two of the included studies reported no significant difference in muscle oxygenation and blood lactate concentration between CG and control groups taken at the flexor carpi radial muscle of national flat-water kayakers24 and vastus lateralis of competitive cyclists,29 with the latter further reporting no significant difference for total hemoglobin level. Goto et al9 showed that wearing full body CG for post-exercise recovery significantly reduced upper arm and thigh circumference after strenuous resistance exercises compared to the control group. Blood lactate levels of semi-professional rugby league players were significantly lower while performing moderate intensity run on the treadmill, but no significant effect was reported when running at high-intensity compared to control group.27 This is interesting as Lovell et al27 is the only study among the eight selected that reported a significant drop in blood lactate concentration after a while following the removal of CG.
Three studies reported no significant difference between skeletal muscle power or force production for highly-trained athletes when performing various exercise protocols wearing CG compared to the control group. Dascombe et al24 found no significant difference between conditions for total work and relative work in well-trained kayakers during a four-minute maximal performance test. This is similar to a past study32 that showed no significant effects of CG to aid sports performances. Collectively, this review suggests that CGs may not have any worthwhile effects on skeletal muscle physiology and performance.
Skeletal muscle recovery: With regard to the effects of using CGs for recovery from athletic performance, previous studies have shown that CGs could be effective in speeding up recovery by reducing perceived muscle soreness and levels of creatine kinase (CK), one of the markers of muscle damage, 24-hours post-ex ercise.10 However, another study32 found reduced muscle soreness only for the CG group 24-hours after the 10 km run but not for the multistage shuttle run performance. Possible explanations for differences in reported recovery outcome variables include variation in the type and applied pressure of the CGs tested as well as the duration of the recovery period that the CGs were worn following exercise. However, these studies did not include a control group thus making the validity of the outcomes contentious.
Four of the studies included in this review reported no significant difference in CK concentration between groups. Only one study reported a significant reduction in blood lactate levels during active recovery after submaximal treadmill test while the others reported no significant different between CG and control group. Goto et al9 reported significant recovery of muscle strength after wearing CG while Duffield et al25 reported no significant effect of CG on other muscle damage markers such as concentrations of CRP and the enzyme aspartate transaminase. Interestingly, one study found a significant reduction in five repetition countermovement jumps 24-hours post recovery in the CG group, although no significant effect was found for other time-related variables and peak force during post-exercise recovery.28 To the best of our knowledge, this was the only study reporting that wearing CG had significantly poorer outcome than the control group. This could possibly have meant that the beneficial effects of CGs, if any, might only last for the first 48-hours or so after recovery. Collectively, the strength of evidence on the effects of CGs on skeletal muscle function recovery after exercise seems weak as well as unclear.
LIMITATIONS
The PEDro scale assessment of the studies was done by the lead author only, which could have introduced an element of bias in this systematic review. Due to the strict eligibility criteria, there was a lack of stronger evidence such as RCT study designs to support this systematic review. Seven of the eight studies used highly-trained athletes as subjects, hence the outcomes maybe not be reflective of the wider population of healthy 18 to 30-years age group with different fitness levels. The combined number of subjects from all eight selected studies was 108, of which only two subjects are confirmed to be female and 56 subjects stated as male. Three of the studies (n=50) failed to specify the gender of their subjects. Judging by their rugby background, they are assumed to be males. Therefore, the total sample size of this systematic review is rather small compared to other systematic reviews.33,34
CONCLUSION
This systematic review aimed at identifying eligible studies to evaluate the effects of CGs on skeletal muscle physiology, performance, and recovery in young healthy adults. In conclusion, this review found that the CGs were effective during exercising for improved blood circulation and moderate intensity runs in term of skeletal muscle physiology-related outcomes. None of the eight studies reported any significant difference between garment conditions for skeletal muscle performance. For post-exercise skeletal muscle recovery, CGs may speed up restoration of muscular strength and clearing of blood lactate concentration when active recovery is employed. Even though most studies reported no significant difference between CG and control groups for most of the skeletal muscle variables, differences do exist between the absolute values measured in the various conditions, although not large enough to be considered statistically different.
Future studies should focus on using recreationally active or sedentary subjects to include the expanse of variation in healthy young adult subjects and fitness levels to better elucidate the effects of CGs on a larger population. More studies need to be done to build on the current body of evidence to determine the effects of CGs on peak power and possibly other skeletal muscle variables during recovery, after the removal of CG. To the best of our knowledge, there are no negative effects of CGs reported till date. Therefore, CGs can safely be worn during or after exercise for individual comfort and belief, despite its currently uncertain physical and physiological benefits related to performance and recovery
CONFLICTS OF INTEREST
The authors declare that they have no conflicts of interest.
FUNDING
This study did not receive any funding.