INTRODUCTION
Digital tomosynthesis (DT) is a unique imaging modality that has been recently developed for general radiography. In clinical practice, it has been researched and used primarily for mammography, where it involves a rotating detector and tube used to image the breast over an arc of up to 60°. The data is then reconstructed and displayed as an image stack that can be viewed as slices, which allows for additional volumetric and depth information to localise any abnormalities.1 Although it has been widely adopted for clinical practice in breast imaging, there is less research on the use of DT in other parts of the body. There exists general radiography equipment that can perform DT however, there are only several units in use in Australia (Davidson R, 2018, unpublished data). Therefore, this literature review will focus on providing an introduction to whole body DT, from its basic parameters to how it is used in each region.
Digital tomosynthesis began as conventional tomography, where the X-ray tube and detector would move in opposite directions over a limited angular range. Images would be acquired on film and only one central plane, called the fulcrum, would be in focus, blurring the anterior and posterior planes. More imaging was required to resolve other planes, resulting in excessive dose and increased examination time, which were barriers to its adoption in clinical practice.2,3 Currently, DT employs a large flat panel detector technique. Rather than having both the detector and tube rotate, only the tube rotates over an angular range (θ) as illustrated in Figure 1.
Figure 1. The Fundamental Principles of Digital Radiographic Tomosynthesis
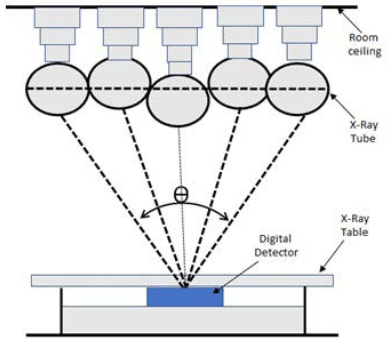
The data is then reconstructed, which allows for a specific plane to be resolved, and volumetric data is provided of a structure at a lower dose than a computed tomography (CT) scan.3
PURPOSE OF THE LITERATURE REVIEW
Research on DT has been conducted extensively in relation to breast imaging, but significantly less for other body regions. The purpose of this literature review is to investigate and identify the major uses of DT in those regions, and its use in clinical practice.
METHODOLOGY
An initial search was conducted through the databases Ovid, MEDLINE, Scopus and PubMed with keywords of DT in conjunction with multiple other terms. Specifically: “chest”, “head and neck”, “orthopaedic”, “abdominal”, “whole body”, “acquisition parameters” and “reconstruction methods”. The results were limited to articles in English that were published in the last five years, and can be seen in Figure 2. A subsequent reference search was conducted to identify additional relevant articles.
Figure 2. Flow Chart Diagram to Illustrate the Method Used to Determine Relevant Literature
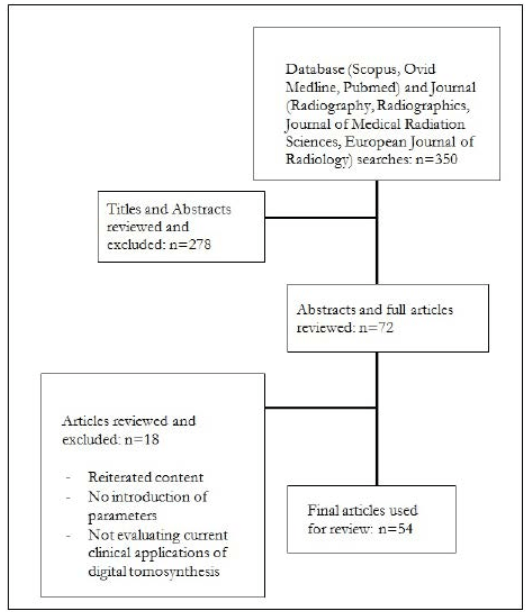
Vendors providing general radiography equipment capable of DT were identified through a search of their websites, specifically General Electric (GE), ShimadzuTM, Siemens, HologicTM, PhilipsTM, Carestream and ToshibaTM.
DISCUSSION
Principles and Parameters
Conventional digital radiography employs a static detector and tube, and produces a single projection. In comparison, DT acquires multiple low dose projections as the X-ray tube performs an arc over the region of interest, and therefore involves different parameters. In clinical practice, DT has been extensively researched and developed for breast imaging, where the gold standard is mammography. However, in denser breasts, detectability can be compromised as the breast tissue can mask any lesions.1,4 Digital tomosynthesis provides a method to overcome this issue, allowing for depth localization without the increased dose from CT.
The same concept is used for DT of the whole body, where applications have been identified primarily in the chest, abdomen, head and neck, and extremities.5 Besides the degree of breast compression, the parameters used for both breast and whole body tomosynthesis remain the same. In Figure 1, the movement of the tube can be seen in both vertical and horizontal applications, the angle or extent of motion is called the sweep angle, and the direction of movement: sweep direction. This would typically be from 20° to 50°, where 50° is ±25° from the centre. The number of projections acquired per movement of tube, calculated by dividing the total number of projections by the sweep angle, is called the projection density. Other important aspects to consider are the distance between the patient’s skin edge to the object of interest, barrier-object distance, and radiation dose. The total dose is calculated by multiplying the dose per projection with the total number of projections.3,6
To produce the viewable image dataset, there are different reconstruction methods that have been developed. Originally, back projection was used to reconstruct the data, where it followed a straightforward implementation method similar to the back projection used with CT. However, this lead to significant blurring and image degradation that further methods were explored.2 Digital tomosynthesis involves a limited arc of the X-ray tube, compared to the revolutions that occur in CT. Therefore the same principles cannot be directly applied in reconstruction; CT relies on having enough sampled data in frequency space, but DT has incomplete sampling where entire regions are missed. This lead to filters being developed to address the blurring and discrepancies in DT specifically, which functioned to force the system to assume a uniform depth response over the range of frequencies obtained.2 Essentially, they are low-pass filters applied in the frequency domain to suppress the high frequency information, and compensate for the sampling issues in tomosynthesis. The other two main methods of reconstruction are matrix inversion reconstruction, and iterative reconstruction. Matrix inversion reconstruction involves applying an algorithm inverse to the blurring that occurs in the data. Iterative reconstruction involves a loop where the projection data is processed through an algorithm, and goes through iterations until it reaches a set criterion. This may be a certain level of noise; therefore, a dataset with higher spatial resolution or sharpness may be obtained through iterative reconstruction. However, it is more computationally demanding compared to the other reconstruction methods, and therefore it is more common to see filtered back projection used for standard DT examinations.7
When interpreting the image set to determine their diagnostic quality, the key metrics are the in-slice resolution and the depth resolution. In-slice resolution refers to the smallest structure that can be resolved in a slice. Digital tomosynthesis uses filtered back projection to reconstruct the data into slices, which is applied in columns perpendicular to the focal path and results in lowered resolution vertically compared to horizontally.3 The type of detector used limits the resolution in the horizontal plane, like in conventional digital radiography. Additionally, noise is dependent on the radiation dose, and can be reduced either by increasing the projections taken, or the dose for each projection.3 For depth resolution, DT involves a limited sweep of the tube and detector, which doesn’t result in full volumetric information, but can provide insight into the depth of structures. With a larger sweep angle, there is greater differentiation per projection, resulting in improved depth resolution.6 The other factors affecting image quality include artefacts, where the main occurrences are blurring, ghosting and ripple artefacts.3,6,8
Currently, there are three vendors that provide DT as an advanced optional application. GE, ShimadzuTM and Fujifilm offer DT with their general radiography systems; the Optima 656 PlusTM, RADspeed Pro Edge and the FDR Visionary SuiteTM respectively. In each system, DT can be used with both the wall-stand and table bucky, allowing for both supine and weight bearing or erect positions.9,10,11 In their DT package, ShimadzuTM offers a metal artefact reduction reconstruction method called T-smart, which creates composites by separately reconstructing the regions with metal and without metal with iterative reconstruction. They also offer DT with the Sonialvision G4, which is a fluoroscopy system with a flat panel detector and removable grid. The tube is connected to the table, and can be angled to produce erect images for procedures such as barium swallows.12
Whole Body Applications
Chest tomosynthesis: Chest tomosynthesis performs imaging over a limited arc, blurring overlying anatomy to improve visibility of the lungs in the coronal plane, highlighting its potential to follow-up known nodules.7 It also has applications in cystic fibrosis,13 tuberculosis,14 and asbestos-related diseases.15
Dose-wise, any examination involving DT requires a scout to plan out the procedure. For the chest, this would involve an initial chest radiograph (0.01 mSv) plus the actual DT (0.12 mSv), resulting in a total dose of 0.13 mSv for the examination.16 This figure varies among the literature; many calculate the effective dose to be from 0.10 to 0.14 mSv,17,18,19,20 with two stating higher values of 0.19 mSv, which may be from using different parameters or having a different patient samples.16,21,22 An example of standard chest tomosynthesis parameters can be seen in Table 1.
Table 1. Standard DT Imaging Parameters for Chest Tomosynthesis23,24,25 |
Tube Voltage
|
Tube Current (mA) |
Sweep Angle |
Projections |
Time (s)
|
100-120
|
0.04 |
30-40
|
50-60
74 (Shimadzu) |
10-12
4.85 (Shimadzu) |
In the detection of lesions and nodules, chest radiographs and CT examinations are typically used, where CT is considered the gold standard for characterizing lesions. However, there is a significant difference in dose between the two modalities. A CT Chest results in a dose of 4-8 mSv, or 1.5 mSv if a low dose examination is selected.7 Standard chest radiography consisting of two projections results in a dose of 0.05 mSv, a postero-anterior projection being 0.01 mSv and a lateral, 0.04 mSv. Low dose CT is the gold standard imaging modality for monitoring lung lesions, but with a radiation dose difference of 0.05 mSv versus 1.5 mSv, alternative imaging modalities such as chest tomosynthesis have been considered.16
The sensitivity and applicability of chest tomosynthesis has been investigated in numerous articles, and whether it is a viable screening tool for at-risk patients. A multi-institutional study from Dobbins et al26 compared chest radiography to chest tomosynthesis and dual energy radiography in the management of pulmonary nodules. This study used five radiologists from different specialties, and found that DT showed significant improvement in the detection of nodules compared to CR, with improved visibility in the lung apices. These results align with those of similar studies,27,28 suggesting its viability for nodule screening. However, DT can result in misinterpretation of extraparenchymal lesions, or solid pulmonary nodules below 6 mm, and should still be referred for a baseline CT if nodules have been detected.19,23,24,29
Despite these applications, chest tomosynthesis has significant limitations that should be considered when setting the correct exposure parameters. As there are multiple high-density structures outside the focal plane such as the ribs, it can be particularly prone to ripple or blurring artefacts. Additionally, chest tomosynthesis uses an acquisition time of around 10-12-seconds on a single breath hold, making it susceptible to motion artefacts which could lead to diagnostic errors in nodule detection. To combat this, using a higher projection density has been recommended, raising the number of projections to 60 with a 30° sweep angle.5
Abdominal tomosynthesis: Compared to chest tomosynthesis, there is significantly less literature concerning abdominal tomosynthesis. In the majority of these articles, the focus is of nephrolithiasis, where the current gold standard is non-contrast CT; it can demonstrate secondary signs of obstruction such as perinephric fat stranding and hydronephrosis. However, radiation dose is a significant concern. It has been shown that patients who have an acute stone episode will have a median of 4 diagnostic imaging studies the year following the occurrence, including 1.7 non-contrast CT examinations.30 For younger patients who have recurrent stones, this would significantly increase their yearly radiation dose and their cancer rate, based on studies from atomic bomb radiation outcomes.30 The dose from a standard non-contrast CT examination has been found to be as high as 9 mSv and 12 mSv for men and women respectively,31 but low-dose non-contrast CT procedures for nephrolithiasis have been introduced, which must be below 3 mSv.30,31,32,33 Although this is a significant reduction, research has been made into alternative imaging to further uphold the as low as reasonably achievable (ALARA) principle.
Conventional radiography, specifically the kidney-ureter-bladder (KUB) projection, and ultrasound are typically used for a patient presenting for potential renal stones, and follow-up imaging post-diagnosis. The detection rates of KUBs have been reported as 45-58%.32 This low value has been attributed to overlying bowel, where a study by Liu et al33 measured the detection rate to be 48.7% (n=66/138) and 66.7% (n=92/138) before and after bowel preparation. Digital tomosynthesis is a promising modality in regards to nephrolithiasis as the data is acquired in one sweep of the X-ray tube, and can be reconstructed to produce different focal zones in the coronal plane. This provides limited depth resolution and can allow for radiologists to scan the kidneys to accurately determine the location of stones. The blurring from DT also removes overlying bowel and aids detection.32 In the same study by Liu et al,33 they found DT to have detection rates of 94.2% (n=130/138) and 96.4% (n=133/138) prior to and post-bowel preparation.
Astroza et al33 investigated the dose delivered by standard KUBs, DT and low-dose CT on a 173 cm tall anthropomorphic phantom weighing 73 kg. The dose for DT was found to be 0.83 mSv, compared to 0.63 mSv for the KUB and 3.04 mSv for the low dose CT. Although these values are from a standard patient size, and do not account for patient variation, abdominal tomosynthesis would be a viable screening modality for non-acute stone detection.32,34 An example of standard parameters can be seen in Table 2, where the patient is imaged supine and over a breath hold.
Table 2. Standard DT Imaging Parameters for Abdominal Tomosynthesis32,35 |
Tube Voltage
|
Tube Current (mA) |
Sweep Angle |
Projections |
Time (s)
|
85
|
630 |
30-40
|
17 slices
reconstructed |
5.5 |
Head and neck tomosynthesis: For the head and neck region, DT has been researched regarding the paranasal sinuses. As with the abdomen, there was significantly less literature available on the topic, with only 3 being within the search range of 2013 to 2018. All 3 pertained to the viability of tomosynthesis as a screening and follow-up tool for sinusitis where the current the gold standard imaging approach is CT or low-dose CT. For this region, the significant concern is the radiation dose to the radiosensitive regions such as the eye lens and the thyroid, especially as CT involves concentric arcs of the detector and tube. This results in a significantly higher dose to the region compared to standard radiography, which encompasses a two image radiographic skull series of a Caldwell and Water’s view. For the eye lens, radiation-induced opacities occur with worsening severity from 0.5-2 Gy; CT, radiography and DT all have doses significantly below that threshold.36,37 However, in populations such as paediatrics, who are ten times more sensitive to ionizing radiation, damage can occur to the eye lens with a cumulative exposure of 250 mGy.36
Yoo et al37 compared sinus radiography and DT in radiation dose, sensitivity and specificity, using CT as a reference standard. The images were interpreted by radiologists with varying familiarity with DT, with no mention of blinding. For sinusitis, the two radiologists, (A and B) were found to have significantly higher sensitivities for DT (A: 96%, n=24/25; B: 92%, n=23/25) compared to radiography (A: 52%, n=13/25; B: 80%, n=20/25) with similar specificities.37 This was corroborated by Machida et al,38 who additionally found high sensitivities and specificities in the frontal, ethmoid, and sphenoid sinuses. In regards to eye lens and thyroid doses, DT results in 0.1 mGy and 0.2 mGy respectively, where CT results in 10-32 mGy to the eye lens, and 0.6-1 mGy to the thyroid. Combined with the higher sensitivity and specificity to radiography, DT of the sinuses is a prospective imaging tool for screening in radiosensitive populations.36
Imaging-wise, the parameters can be seen in Table 3. With a slightly higher dose, sinus tomosynthesis has increased sensitivity and specificity of the maxillary, frontal, ethmoid and sphenoid sinuses, and has been suggested as a screening tool to replace radiography. However, it is susceptible to motion artifacts,37 and has limited literature available on the topic, with the most recent article being a pilot study published in 2013.38 Despite its potential applications in radiosensitive populations, it has yet to influence clinical practice.
Table 3. Standard Parameters for Sinus Tomosynthesis36,37,38 |
Tube Voltage
|
Tube Current (mA) |
Sweep Angle |
Projections |
80-200 |
1 |
40 |
60
|
Orthopaedic tomosynthesis: In orthopaedics, DT can more clearly define complex fractures and can rule out ambiguous cases, or monitor degenerative disease which can reduce the need for CT. In a clinical setting, as it is offered as an advanced modality option from major vendors, it could serve as a bridging modality to save both radiation and in-department time for the patients in radiographic examinations.39 This is reflected in the cost, where studies have described lower per-patient diagnostic imaging costs upon implementation, as well as a reduced need for CT.40
The imaging parameters in orthopaedics vary with each region, where the standard sweep angle set would be around 40°. The kVp and mAs are dependent on the body region; for the wrist or foot, this would be 50-60 kVp and around 0.6 mA.41,42,43
CONCLUSION
Digital tomosynthesis is a promising imaging modality that acquires multiple low dose projections over a limited arc of the X-ray tube, and produces a stack of slices in the acquisition plane using image reconstruction. Through this, it provides depth resolution and reduces the degree of obscuration by overlying structures, improving the sensitivity compared to conventional radiography at a significantly lower dose to CT. Despite being prone to artefacts, it has been shown to be effective for surveillance and identification of occult fractures. Currently available as an add-on to conventional radiography equipment, DT has the potential to reduce the radiation burden, cost and time in department of patients who require continual imaging. However, large-scale studies demonstrating statistically significant results are required to validate its place as an intermediate modality in clinical practice.
CONFLICTS OF INTEREST
The authors declare that they have no conflicts of interest.