INTRODUCTION
I t is clear that ideal body weight and composition are related to allcause mortality, health, and longevity in healthy and non-healthy populations including breast cancer survivors.1,2 Additionally, there is a strong inverse relationship between survival rates related to levels of body fat as well as the development of comorbidities like osteoporosis in cancer survivors.3,4,5 Knowing the criterion measure of body composition that confers a protective advantage to survival and how to interpret the value based on the assessment tool has significant health ramifications. Errant determination of body composition may lead to a false assumption of risk and subsequent poor energy balance strategies. In the numerous studies on body composition of cancer patients, multiple methods and values have been reported making it clear that measures of body composition including percentage of fat, vary considerably from each method.6,7
There exist numerous methods to estimate total body composition in situ including water dilution, hydrodensity, pthlesymography, bioelectrical impedance, and anthropometry. Each method has benefits and limitations and range considerably in reliability, validity, and associated costs.8 However, it is clear that dual X-ray absorptiometry (DXA) is far superior in that actual compartments of fat, fat free mass, and bone can be measured precisely and is now considered a gold standard in body composition analysis.9,10,11 Outside of the clinical setting, typical rehabilitation centers, and/or training studies, body composition is routinely estimated from measures of subcutaneous fat and a double layer of skin. These measures are then used to estimate body composition based on reference data. Skinfold measurements are relatively easy to perform, have moderate validity, and are very cost-effective.
A question of interest is, how do different body composition assessment methods compare for a rather select and active population of older female breast cancer survivors? Therefore, the purpose of this study was to compare body density and percentage of fat measured using DXA versus estimated measures of percentage of fat estimated from skinfold thickness. It was hypothesized that body density measured by DXA would be significantly lower (and percentage of fat and fat mass significantly higher) when compared to body density (and percentage of fat) estimated from skinfold measures. Additionally, using the functional capabilities of the DXA, we were able to compare intra-subject bone mineral content of the upper and thoracic region in order to compare bone mineral content of the tumor side versus the non-affected side in an attempt to determine if there were bilateral differences in body structure.
MATERIALS AND METHODS
Forty breast cancer survivors (57.0±9.3 y, range: 31-73 y) from a local competitive Dragon Boat team provided written informed consent and provided signed physician (either oncologist or primary care) assent to participate in the project. The project was approved by the Institutional Review Board (IRB) of the University of Indianapolis, #01070. This study is part of a larger study evaluating the physiological profiles of a group of very active breast cancer survivors.
Of the 40 women, 37 were Caucasian and 3 were black (not of African descent), and all subjects had previously been diagnosed with breast cancer. Body composition anthropometry included skinfold measures at nine sites (triceps, biceps, subscapular, mid-axilla, iliac crest, supraspinal, abdominal, thigh, and medial calf) along with anthropometric measures of bone breadths of the humerus and femur, and six circumference measures (upper arm relaxed and flexed, waist, hips, mid-thigh and calf). The extensive list of body measures was done as part of a larger project to compare active breast cancer survivors from different geographical regions, and not all measurements were used to calculate body density nor percentage of fat in this study.
Subjects differed in treatment type (chemotherapy, surgical intervention, and radiation exposure), time from diagnosis (0- 25 y), time in treatment (2-months-10 y), and years of experience on the team (5.4±3.7, range< 1-12 y). Following reconstructive surgery, 13 of the 40 subjects had either saline or silicone prosthetic implants inserted.
Stature was measured with a wall-mounted stadiometer (Seca 217, Hamburg, Germany) and was recorded to the nearest 0.1 cm. Weight was measured with a digital scale (Tanita TBF300A, Japan) to the nearest 0.1 kg. Body mass index (BMI) was calculated and recorded as kg*m-2. Bone breadths were made with a small sliding caliper (Martin type, anthropometer) and all circumference measures were made with a fiberglass tape measure fit with a Gullick handle (GRAFCO, Camalò TV, Italy), and measured to the nearest millimeter. Body composition was estimated with skinfold measures using a Harpenden skinfold caliper, and all measures were made on the right side of the body according to Lohman et al.12 Age and sex-specific equations were used to estimate body density (Bd) and/or percentage of fat from skinfold measures, and are presented in Table 1.13 The same technician made all measures and has a reported technical error of measurement (TEM) between 0.12-0.60 mm.
Table 1. Regression Equations Used to Determine Body Density and Percentage of Fat in Females |
Author
|
Equation |
Jackson et al13 |
Bd=1.09609500.0006952(x)+0.0000011(x2)-0.0000714(age)
X=sum of triceps, abdominal, suprailium, thigh skinfolds
|
Lohman et al12
|
Percentage fat=(503.3/Bd)-459.2 |
Jackson et al13 |
Percentage fat (3 sites)=0.41563*(x)-0.00112* (x)2 +(0.03661*age)+4.03653
X=sum of triceps, suprailiac, abdominal skinfolds
|
Jackson et al13
|
Percentage fat (4 sites)=0.29669*(x)-0.00043*(x)2 +(0.02963*age)+(1.407)
X=sum of triceps, abdominal, suprailiac, thigh skinfolds
|
Bd=body density (g*cc-1), all skinfold measures are made the nearest millimeter |
BCS were scanned using a GE Lunar EnCore based xray densitometer,and body composition was determined from GE lunar software (2014).14 Prior to all scans, the densitometer was calibrated in accordance with the manufacturer’s instructions and passed all quality assurance tests. All subjects were measured in the supine position, wore minimal clothing, and removed all metal objects. Scans were on average 6-12-minutes in duration and radiation exposure was approximately 3-6 μGy. Total and regional bone mineral densities were recorded. Tissue was defined as fat and lean tissue weight in grams without bone, and tissue percentage fat was defined as fat (gm)*tissue (gm)-1. Regional percentage fat was defined fat (gm)*(tissue (gm)+bone mineralcontent-1).
Statistics
All descriptive statistics are reported as means and standard deviations with ranges where appropriate. In order to compare body composition from DXA and anthropometry, one-way analysis of variance (ANOVA) with Dunn’s post-hoc testing was conducted with an a priori probability set at 0.05. Additionally, t-tests were used to compare bilateral bone mineral content of the upper thoracic region. Pearson product moment correlations were made to determine the strongest association among DXA and prediction equations. All statistics were computed using statistical package for the social sciences (SPSS) (IBM SPSS Statistics for Windows, Version 25.0).
RESULTS
Forty (n=40) breast-conserving surgery (BCS) with a cancer diagnosis ranging from stage 0-4 completed testing. Demographic characteristics and anthropometry measures including and upper right and left thoracic region bone mineral density (BMD) for the sample are presented in Table 2. Skinfold measures for all sites, bone breadths, and circumferences are presented in Table 3, and may be useful for future comparisons.
Table 2. Descriptive Characteristics of Female Breast Cancer Patients (N=40) |
|
Mean±Std
|
Range (Min-Max)
|
Age (y)
|
57.0±9.3 |
31-73
|
Height (m)
|
1.64±0.06 |
1.53-1.75 |
Weight (kg) |
77.7±15.3 |
57.4-129.6
|
BMI (kg*m-2)
|
28.8±5.2 |
21.0-43.3 |
BMD total body(gm*cc-2) |
1.154±0.097 |
0.989-1.315
|
BMD upper thoracic cancer(gm*cc-2)
|
0.971±0.198 |
0.689-1.391 |
BMD upper thoracic healthy(gm*cc-2) |
0.988±0.190 |
0.722-1.531
|
Years since diagnosis (y)
|
8.6±5.4 |
<1-26 |
Post-Menopausal (n) |
38 of 40 |
|
Years on team (y)
|
5.4±3.7 |
<1-10 |
Active Prior to diagnosis (n) |
31 of 40 |
|
Treatment Type (n) |
Surgery (11) [7 mastectomies, 14 double mastectomies, 14 lumpectomies]
Radiation (8)
Surgery+Chemotherapy (7)
Surgery+Chemotherapy+Radiation (10)
Surgery+Radiation (3) Chemotherapy+Radiation (1)
Not Identified (1)
Number with implants (n) (13) |
Table 3. Anthropometry of Active Breast Cancer Survivors (N=40) |
Measure
|
Mean±Std |
Range (Min-Max)
|
Triceps 24±6 13-33
|
24±6 |
13-33 |
Subscapular 21±8 7-34 |
21±8 |
7-34
|
Biceps 15±7 4-30
|
15±7 |
4-30 |
Iliac Crest 23±8 7-36 |
23±8 |
7-36
|
Supraspinal
|
20±8 |
6-38 |
Abdomina |
24±7 |
11-39
|
Thigh
|
28±7 |
8-40 |
Calf |
21±7 |
6-38
|
Mid-axilla
|
17±7 |
5-33 |
SSK (9 sites) |
192±53 |
95-303
|
Arm circumference,relaxed
|
29±4 |
20-39 |
Arm circumference,flexed
|
31±4 |
26-40
|
Calf max |
37±5 |
30-59
|
Mid-thigh
|
52±9 |
33-92 |
Humerus |
7±0 |
6-7
|
Femur
|
11±1 |
9-13 |
Waist |
86±12 |
68-118
|
Hips
|
103±11 |
86-139 |
Waist-to-Hip ratio |
0.8±0.1 |
0.7-1.0
|
All skinfold measures were made the nearest millimeter (mm). Circumferences and bone breadths were measured to the nearest centimeter (cm). All measures made according to Lohman et al12 |
An analysis of variance (ANOVA) on these scores yielded significant variation among the methods to determine percentage fat. A post-hoc Dunn’s test showed that percentage of fat determined from DXA, 41.1±6.0%, was significantly higher than percentage fat calculated with the three anthropometric equations, 28.8±4.9, 22.1±4.1, 31.8±9.4% (Table 4). Additionally, body fat determined using the Jackson et al13 4-site equations (22.1±4.1) was significantly lower than the two remaining estimates of body fat (28.8±4.9 and 31.8±9.1). The highest correlation between the DXA and three site determination was r=0.81. BMD of the upper thoracic region was similar and independent of side of breast cancer diagnosis (right 0.971±0.198 vs. left 0.988±0.190 gm*cc-1, p=0.2, non-significant). Effect size was calculated using pooled averages and standard deviations, and resulted in a value of 2.26 (Figure 1).
Table 4. Body Composition of Active Breast Cancer Survivors (N =40) |
Measure
|
Mean±Std |
Range (Min-Max) |
p
|
% fat from DXA
|
41.4±6.0* |
28.8-50.7 |
0.000 |
% fat with three skinfolds |
28.8±4.9** |
19.1-36.2 |
0.34
|
% fat with four skinfolds
|
22.1±4.1*** |
12.9-29.2 |
0.004 |
% fat from Bd and Lohman equation |
31.8±9.4** |
16.8-47.7 |
0.34
|
* significantly different from all other equations (p<0.001)
**non-significant difference, p=0.34
***significantly lower than other two skinfold measure equations, p=0.004 |
Figure 1. Pearson Product Moment Correlation between DXA and Three-site Skinfold Measures, r=0.81
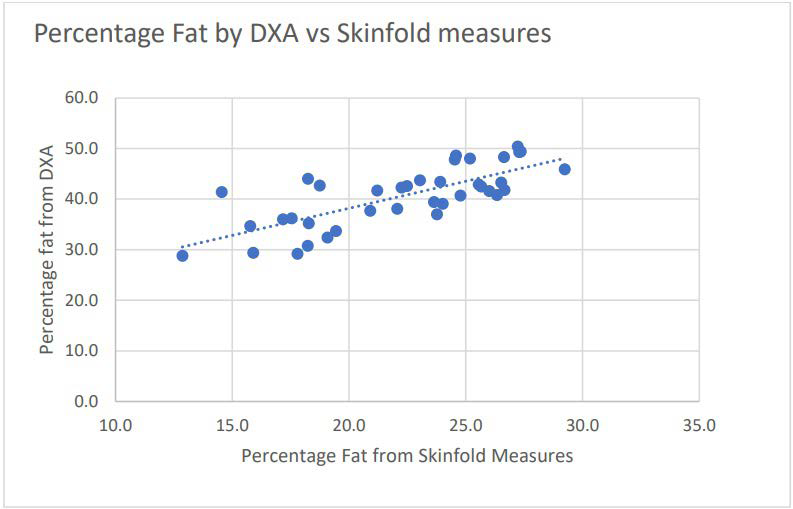
DISCUSSION
The purpose of this study was to compare body composition (body density, fat mass, fat free mass, percentage of fat) measured using DXA versus body density estimated from measures of subcutaneous fat (skinfold measures), and subsequently used to determine percentage of fat. While repeated measures designed for body composition using one method would provide useful feed back about training efficacy, using only anthropometry to estimate body fat would have resulted in clinically erroneous results. Ideal body weight or weight management in athletics or cancer survivorship is equally important, and therefore having a more valid measure of fat mass is critical.1,15,16 It was hypothesized that body density would be significantly lower when measured by DXA (and percentage of fat and fat mass would be significantly higher) when compared to body density (and percentage of fat) determined from skinfold measures. The results of this study confirm our hypothesis and are in line with other researcher results which indicated percentage of fat determined by DXA is significantly higher than that determined with skinfold thickness measures alone.11,17 In this study, reporting body composition values obtained from anthropometry would have resulted in a “very large” effect size (ES=2.26).
Finally, using the functional capacity of the DXA, we were able to compare intra-subject bone mineral content of the upper and thoracic region in order to compare bone mineral content of the cancer vs. non-cancer affected sides as part of a broader research question. It was hypothesized that tumor load and treatment may have had a differential effect on upper body integrity. This was not supported with our data as bone mineral content did not differ based on side of cancer diagnosis. The reason may be that in this rather small group of active BCS, the demands of Dragon Boat racing and bilateral training (paddling both left and right sides) provided a sufficient stimulus to maintain bone mineral content on both sides of the upper body.
The correlation between DXA and percentage of fat determined from the regression equation using three skinfolds was 0.81 indicating a strong positive relationship. However, there was, on average a 12% difference in percentage of fat between the two methods. The coefficient of variation, 0.64 indicated that 36% of the variance between the two methods is unaccounted.
Reasons for the 36% difference in calculating percentage of fat using these two methods included the following. Direct measure of fat and fat-free mass (minus bone mineral content) which cannot be accounted for in the two-compartment model typically used in skinfold analysis. A second variable contributing to the variance between the two measures based on manufacturer’s information, is that prosthetics (density and volume) must be accounted for. In the GE Lunar software program, saline prosthetics are calculated as body water while silicone prosthetics are included as a fat mass. Size and volume of the prosthetics were not available for this study. Lastly, anthropometry is subject to technician error (even with low TEM), and results in a minimal standard of error of 3% which may have contributed to overall variance between the methods. Finally, using multiple regression equations based on cadaver studies assumes uniform density of fat and fat free mass which has been clearly shown not to be accurate.7 Additionally, bone, skin, and internal organs are all considered part of FFM in the two-compartment model which results in overestimation of fat free mass while underestimating total fat mass.
DXA has effectively replaced hydrodensitometry as the gold standard of body composition analysis and is routinely used by the National Institutes of Health (NIH) and Centers for Disease Control and Prevention (CDC) for assessing body composition.9 However, not all entities are able to assess body composition using this method. As a result, clinicians who report body composition determined solely from anthropometry may be providing significantly erroneous values. Future research will necessitate comparing a large population of healthy women, and women diagnosed with cancer to determine any variance in population demographics. Additionally, adjustment to existing regression equations used to predict percentage of body that use DXA as a criterion measure should be reported for both healthy and diseased individuals.
CONCLUSION
The estimation of body fat in a group of active breast cancer survivors was significantly higher when measured by DXA vs. that estimated from anthropometry. If clinicians need to provide valid assessment of body composition for this population, use of skinfold measures are not supported. However, if the only assessment of body composition can be completed using anthropometry, reporting sum of skinfolds and using specific age- and sex-specific regressions for an active population must be considered.
Practical Implications
• Percentage of fat determined from DXA was significantly higher in a group of active breast cancer survivors compared to measures obtained from skinfolds.
• If anthropometry is used to describe body composition, consider reporting sum of skinfolds.
• Prosthetics (type and volume) may have a significant impact on body composition determination in breast cancer survivors.
INFORMED CONSENT
Forty breast cancer survivors (BCS) (57.0±9.3 y, range: 31-73 y) from a local competitive Dragon Boat team provided written informed consent and provided signed physician (either oncologist or primary care) assent to participate in the project.
INSTITUTIONAL REVIEW BOARD
The project was approved by the Institutional Review Board of the University of Indianapolis, #01070.
AUTHOR CONTRIBUTIONS
RK, research design, collected and analyzed all data, wrote manuscript. BH and SC collected all data, reviewed final manuscript..
CONFLICTS OF INTEREST
The authors declare that they have no conflicts of interest.