INTRODUCTION
Edema is defined as a grossly swollen condition caused by an excessive amount of fluid in inter tissue spaces.1 The fluid is stored in the fat layer, mainly in the subcutaneous tissue. Edema can be caused by anything from a routine condition to a serious life-threatening disease, such as heart failure or insect bites.1,2,3 To quickly determine whether edema is caused by a serious disease, it is desirable to establish a safe, accurate, and rapid diagnostic method. In addition, this inexpensive and simple method is expected to be applicable to a large number of people.
Currently, the degree of edema is mostly diagnosed using the indentation test.4 The indentation test determines the degree of edema by applying finger pressure to the edematous area from the outer skin and measuring the recovery time of the indented skin. This method is based on an artificial judgment of a medical doctor. Thus, depending on the skill and experience of the medical doctor, individual differences in diagnostic results may occur.
In addition to the indentation test, other methods for measuring edema have also been proposed and implemented. These include edema meter,4,5 bioimpedance,6,7 X-ray,8 computed tomography (CT),8 and magnetic resonance imaging (MRI).8
The edema meter measures the volume of the foot using a water tank. Water is not hygienic, and the preparation of water tanks and scales is time-consuming.
The bioimpedance method measures the resistance of a living body by applying an electric current that is sufficiently weak to cause no abnormalities in the body. This method has the advantage of being able to distinguish edema from obesity by utilizing the difference in resistivity between water and fat; however, it takes time to provide diagnostic results because the measurement is conducted over time.
X-ray and CT images can show differences in soft tissue and tissue structure more accurately than ultrasound images; however, they are not safe for the human body owing to the effects of radiation exposure. In MRI, images are produced by observing the inhomogeneity of the local magnetic field created by various structures in the body in a strong static magnetic field created by a large electromagnet inside the device and classifying them as individual tissues. This method is fast and safe; however, it is expensive owing to the large size and stationary nature of the equipment.
Therefore, it is desirable to establish a simple, inexpensive, safe, accurate, and rapid method for diagnosing edema. To satisfy these requirements, we conducted basic investigations for the diagnosis of edema using ultrasound. Few studies have been conducted on the diagnosis of edema using ultrasound.9,10,11,12,13,14,15,16,17 B-mode imaging with ultrasound diagnostic devices has been studied by the authors’ group and others.18,19,20,21 However, ultrasound diagnostic devices are expensive and challenging to operate. In other words, operation and diagnosis are difficult and require training and experience; hence, the diagnostic results of the devices vary from person to person. Ultrasound diagnostic devices produce images based on the velocity and attenuation of ultrasound waves. In most ultrasound diagnostic devices, sound velocity is predetermined. However, because the area where edema occurs is composed of fat and water, the structure and condition of the edema are likely to change, thus, the velocity of sound is unstable. In addition, the sound velocity varies significantly depending on the measurement area and location. Attenuation is significantly affected by the contact between the ultrasound probe and the human body. In other words, we believe that a diagnosis based on the velocity and attenuation of ultrasound is unstable, and a quantitative diagnosis of edema is difficult.
In other words, it is difficult to diagnose edema quantitatively using only B-mode images from conventional ultrasound diagnostic devices. Therefore, the purpose of this study was to diagnose edema using ultrasound, an easy, inexpensive, safe, accurate, and rapid method. Our goal was to diagnose edema quantitatively by focusing on the propagation time waveform information of the ultrasound wave rather than using the B-mode images of the ultrasound diagnostic devices. The time waveform of ultrasonic wave propagation varies significantly depending on the medium in which it propagates.14,22,23 Using this principle, significant results have been achieved in the medical and industrial fields.24,25,26,27 In this study, we focused on the ultrasonic propagation time waveform to apply this principle. The water-to-fat ratio varies with the degree of edema. When ultrasound waves propagate through a suspended medium, such as a mixture of water and fat (e.g., edema), the propagating waveform of the ultrasound and its frequency analysis information are characteristic.28,29,30,31
In this study, we focused on the ultrasound propagation time waveform to apply this principle. The targets of the diagnosis were the lower limbs, where edema is frequently observed.15,16,17 The front surface of the tibia was chosen as the test site because it is thought to be more affected by water and fat and has fewer blood vessels and muscles.
A fundamental study was conducted focusing on the propagation waveform information of the ultrasonic waves emitted from a self-made ultrasonic transducer. The lower extremities of a healthy person and a person with edema caused by heart disorder were examined.
First, the ultrasound propagation time waveform was observed with and without edemas. From these results, we estimated the propagation distance based on the velocity of the sound in water. This distance was defined in this study as the degree of edema, ED.
The presence of an edema was assessed using the standard deviation of the ED and ED. We also studied the results of the frequency analysis of the ultrasound propagation waveform. Based on these results, we made some discussions and suggestions regarding the location and method of measurement.
EXPERIMENTS
The experimental setup is illustrated in Figure 1. A photograph of the experimental setup is shown in Figure 2. A custom-made polyvinylidene difluoride (PVDF) ultrasonic transducer, which used PVDF piezoelectric films, was used to send and receive ultrasonic waves. A PVDF piezoelectric film is a thin film that can perform mutual conversion between electricity and pressure. Electrical signals were inputs from the pulsar-receiver to the PDVF transducer, and ultrasonic signals were irradiated onto the measurement area, which was the lower limb. The reflected ultrasonic signal from the measurement area was received by the PDVF transducer, converted into an electrical signal, and measured using an oscilloscope.31,32 The transducer consisted of a brass cylinder. The diameter of the transducer was approximately 10 mm. The thickness of the piezoelectric film was approximately 40 µm. The transducer was calibrated using a needle-type hydrophone (HY05N) manufactured by Toray Engineering Co. The pulser-receiver (Olympus 5072PR) was used as the pulser receiver. The pulse signal from the pulsar-receiver was applied to the transducer and ultrasonic pulses were applied to the lower limbs. Ultrasonic waveforms reflected by the fascia and bones were received by the transducer. The received signals were captured using an oscilloscope (TDS1001B, Tektronix). An ultrasound gel was applied between the transducer and epidermis of the lower limb to prevent air bubbles and other particles from entering. Figure 3 shows the measurement locations. Four measurement points were set at 50 mm intervals on the anterior surface of the tibia of the lower limbs, with and without edema. As shown in Figure 3, from the top, we defined the A, B, C, and D measurement points. At each measurement point, the measurement was performed three times without changing the measurer to reduce the influence of artificial errors in the contact angle and contact pressure of the transducer.
Figure 1. Experimental Summary
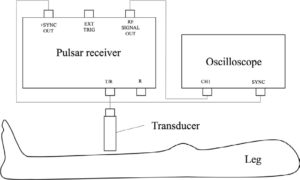
Figure 2. Actual Experimental Scene
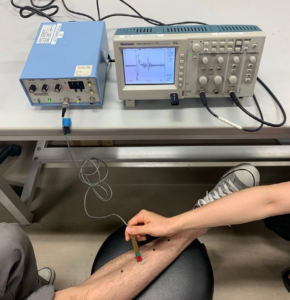
Figure 3. Measurement Points in the Lower Limb Edema
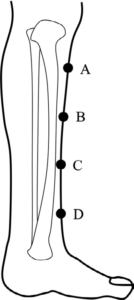
EXPERIMENT RESULTS AND DISCUSSION
Figure 4 shows a schematic of the diagnosis of the inside of the lower limb.33 A fraction of the ultrasonic waves emitted from the ultrasonic transducer (P1) was reflected by the epidermis. Other ultrasonic waves penetrated the epidermis and endothelium. The waves were then reflected by the fascia and bones with high acoustic impedance (P2). The waves then followed their original paths and were received by the ultrasonic transducer.
Figure 4. Schematic Diagram of Internal Tissues in the Lower Limb
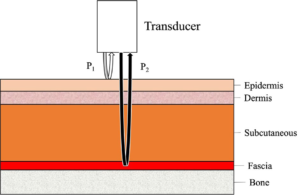
Figure 5 illustrates the propagation waveform using typical measurement results. This figure shows the measurement results at measurement point A for a person without edema. The measurement results at point A for a person with edema are shown in Figure 6. These figures can be explained as follows: The horizontal axis represents time, and the vertical axis represents sound pressure. At time zero, the ultrasonic transducer emitted an ultrasonic wave. As shown in the figure, the pulse at P1 was considered to be the wave reflected from the epidermis. P2 was a reflected wave from a high acoustic impedance region, such as the fascia. The time of the positive maximum of the P1 pulse was defined as t1, and that of the positive maximum of the P2 pulse was defined as t2. The propagation time from the epidermis to the fascia was calculated using the measured ultrasound propagation time waveform. The propagation distance was estimated from the propagation time based on the velocity of sound in typical water.34 The propagation times were t1 and t2, as defined above. In this study, this distance was defined as the value of ED (mm), which represents the degree of edema, for the quantitative diagnosis of edema. The ED is the distance from the epidermis to the fascia, which was calculated based on the ultrasound propagation. Equation (1) was used to calculate, t is the propagation time (s), and v is the sound velocity (m/s). The velocity of sound is 1522 m/s in water at 36 °C.34
Figure 5. Ultrasound Propagation Waveform of a Leg without Typical Edema
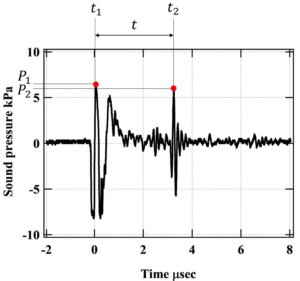
P1 is the maximum value of positive sound pressure in the wave reflected from the surface.
The time of the sound pressure of P1 is t1.
The time of the sound pressure P1 is t1.
P2 is the maximum value of the positive sound pressure of the reflected wave from an area with high acoustic impedance, such as the fascia.
The time of the sound pressure of P2 is t2.
Figure 6. Ultrasound Propagation Waveform of a Leg with Typical Edema
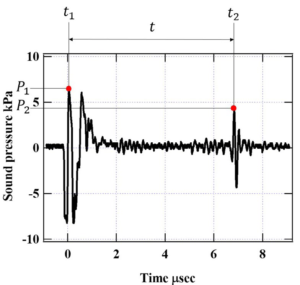
P1 is the maximum value of positive sound pressure in the wave reflected from the surface.
The time of the sound pressure of P1 is t1.
The time of the sound pressure P1 is t1.
P2 is the maximum value of the positive sound pressure of the reflected wave from an area with high acoustic impedance, such as the fascia.
The time of the sound pressure of P2 is t2.
The ED is expressed by the following equation (1):
ED=(t2-t1)×1522×103 ÷2 ————————(1)
In Figure 5, t1 and t2 are 0.0520 and 3.25 µs, respectively, and substituting them into Equation (1) results in an ED of 2.43 mm. Similarly, in Figure 6, t1 and t2 are 0.0520 and 6.83 μs, respectively, which result in an ED of 5.15 mm. Figure 7 shows the results without edema for the third time at each measurement point, and Figure 8 shows the results with edema for the third time at each measurement point. Comparing these figures, it can be seen that the ED for a person without edema was shorter. Comparing these figures, it can be seen that ED is shorter in the absence of edema. These results are similar to those reported by Yanagisawa et al20 and Ikuta et al.21
Figure 7a. Third Propagation Waveform at Measurement Point A for a Lower Limb Without Edema
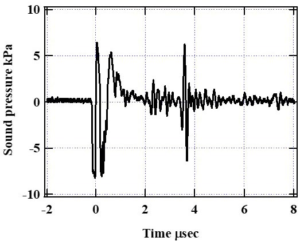
Figure 7b. Third Propagation Waveform at Measurement Point B for a Lower Limb without Edema
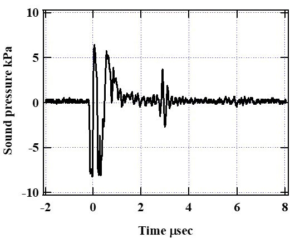
Figure 7c. Third Propagation Waveform at Measurement Point C for a Lower Limb without Edema
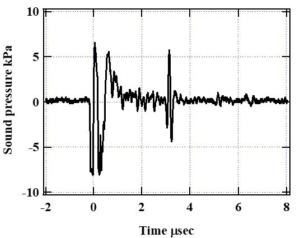
Figure 7d. Third Propagation Waveform at Measurement Point D for a Lower Limb without Edema
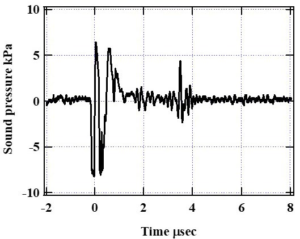
Figure 8a. Third Propagation Waveform at Measurement Point A for a Lower Limb with Edema
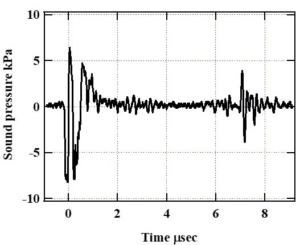
Figure 8b. Third Propagation Waveform at Measurement Point B for a Lower Limb with Edema
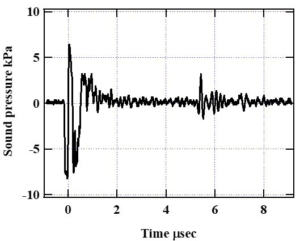
Figure 8c. Third Propagation Waveform at Measurement Point C for a Lower Limb with Edema
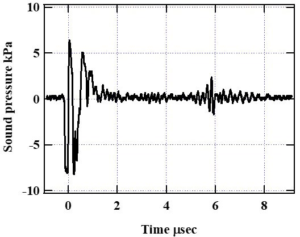
Figure 8d. Third Propagation Waveform at Measurement Point D for a Lower Limb with Edema
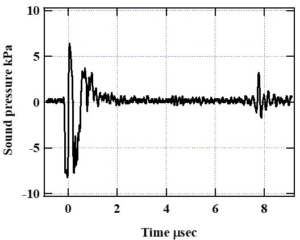
The EDs of the ultrasonic propagation time waveforms of the measurement results for the other conditions were calculated in the same manner. The results are summarized in Tables 1 and 2. Table 1 shows the cases without edema, and Table 2 shows the cases with edema. As described in the experiment, we performed three measurements at each of the four measurement locations. The mean and standard deviation of the three measurements are shown in each Table.
Table 1. Results of Three Measurements of ED at Four Measurement Points in the Absence of Edema. The Mean and Standard Deviation (SD) of the Measurement Results are Shown |
(unit: mm) |
Number of Measurments
|
Times |
Mean |
SD
|
1
|
2 |
3
|
A
|
2.43 |
2.53 |
2.69 |
2.55 |
0.0115 |
B |
2.38 |
2.41 |
2.16 |
2.32 |
0.0124
|
C
|
2.69 |
2.55 |
2.34 |
2.53 |
0.0207 |
D |
2.89 |
2.89 |
2.62 |
2.80 |
0.0162
|
Table 2. Results of Three Measurements of ED at Four Measurement Points in the Presence of Edema. The Mean and Standard Deviation of the Measurement Results are Shown |
(unit: mm) |
Number of Measurments
|
Times |
Mean |
SD
|
1
|
2 |
3
|
A
|
5.15 |
6.36 |
5.34 |
5.62 |
0.282 |
B
|
4.05 |
3.56 |
4.09 |
3.90 |
0.0581 |
C |
5.52 |
5.44 |
4.40 |
5.12 |
0.260
|
D |
5.80 |
5.56 |
5.87 |
5.79 |
0. 00550
|
The cases without edema are explained using Table 1. At measurement position A, the ED showed slight variation among the three measurements. Similarly, for the measuring positions B, C, and D, the ED changed little over the three measurements. The maximum average ED was 2.80 mm, which was obtained at measurement position D, and the minimum average ED was 2.32 mm, which was obtained at measurement position B. The standard deviation of the ED, which represents the variation, was the largest at 0.0207 mm at measurement position C and the smallest at 0.0124 mm at measurement position D. Depending on the measurement position, the standard deviation of the ED showed little variation.
The cases with edema are explained in Table 2. At measurement position A, the ED was found to exhibit a large variation among the three measurements. Similarly, the ED at measurement position C varied substantially among the three measurements. At measurement positions B and D, the variations in ED were small. The average ED differed according to the measurement position. The maximum average ED was 5.79 mm, which was obtained at measurement position D, and the minimum average ED was 3.90 mm, which was obtained at measurement position B. The standard deviation of ED, which represents the variation, was largest at 0.282 mm at measurement position A and smallest at 0.00550 mm at measurement position D.
Next, we compare the results of the measurements with and without edema.
Regardless of the measurement position, the ED was larger, on average, when there was edema than when there was no edema. The average ED was greater than 4 mm for all measurements in persons with edema. The EDs obtained in all measurement positions were greater than 5 mm, except in position B. In contrast, the mean ED for all measurements in persons with edema was approximately 2.5 mm. Overall, the mean ED for cases with edema was approximately twice that for cases without edema. These results are similar to those reported by Yanagisawa et al20 and Ikuta et al.21
In terms of standard deviation, the standard deviation of the ED for the cases without edema for all measurement positions was small (0.02 mm). However, the standard deviation of the ED for cases with edema differed depending on the measurement position. The standard deviations of the ED for the cases with edema were large at measurement positions A and C, which were greater than 0.2 mm. The standard deviation of the ED at position B was smaller than those at A and C, but larger than that at D. The standard deviation of the ED at measurement position B was 0.0581 mm. The standard deviation of the ED at measurement position D was small, which was 0.00550 mm. Measurement positions A, B, and C were affected by the edema and were considered soft because they contained water or other liquids. Therefore, the composition of the measured object changed when the sensor for measurement was touched or released. It is believed that the proportion and arrangement of water and fat in the object changed; thus, the standard deviation was large. At measurement position D, the effect of the edema was high, and the sample was stiff. Measurement position D was the most strongly affected by gravity and is the location where the effect of edema was strongest.15,16,17 Therefore, the composition of the measured object hardly changed when the sensor was touched or released; thus, the standard deviation was not large.
The measurement positions and standard deviations of the ED are discussed. Comparing the standard deviations of the ED for cases with and without edema, the standard deviation of the ED at measurement position D was small regardless of the presence or absence of edema.
Fast fourier transform (FFT) analysis, which is a frequency analysis that allows a more detailed analysis of information on the propagation time waveform of ultrasound, was performed. FFT analysis is a fast method for frequency analysis of time-series signals and is often used in the field of medical ultrasound.35,36,37 Frequency analysis was performed for 1.6 µs after the P1 pulse, the reflected wave from the epidermis, as shown in Figure 5. The Hanning window was used as the window function for frequency analysis. For the time series data, Δt was set to 6.4 µs, and the number of data was set to 1600. We proceeded with the analysis both in the case of edema and in the case without edema. The measurement positions are A, B, C, and D. The time waveforms of the third ultrasound propagation at all measurement positions were analyzed. The results of the frequency analysis for the cases with edema are shown in Figure 9. The results of the frequency analysis for the cases without edema are shown in Figure 10. The horizontal axis represents the frequency and the vertical axis represents the sound pressure.
Figure 9a. Third Frequency Response at Measurement Point A for a Lower Limb without Edema
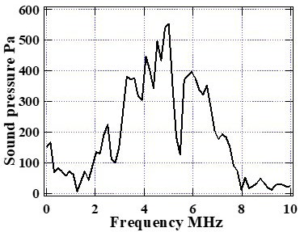
Figure 9b. Third Frequency Response at Measurement Point B for a Lower Limb without Edema
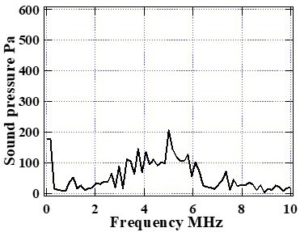
Figure 9c. Third Frequency Response at Measurement Point C for a Lower Limb without Edema
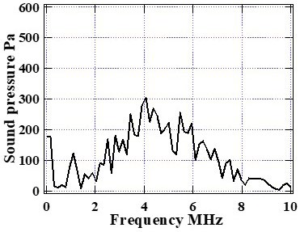
Figure 9d. Third Frequency Response at Measurement Point D for a Lower Limb without Edema
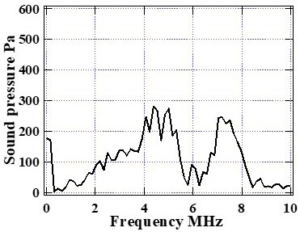
Figure 10a. Third Frequency Response at Measurement Point A for a Lower Limb with Edema
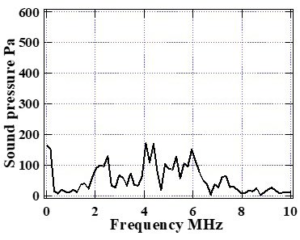
Figure 10b. Third Frequency Response at Measurement Point B for a Lower Limb with Edema
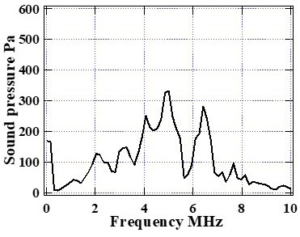
Figure 10c. Third Frequency Response at Measurement Point C for a Lower Limb with Edema
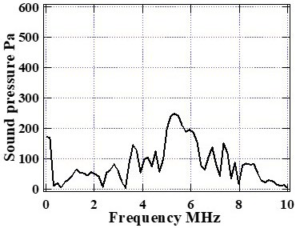
Figure 10d. Third Frequency Response at Measurement Point D for a Lower Limb with Edema
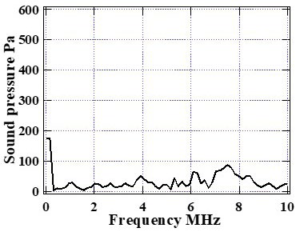
From the results of the frequency analysis, the frequency response, shape of the frequency spectrum, and peak values are discussed. There were no significant differences in the shapes and peak values of the frequency spectrum at measurement positions A, B, and C, depending on the presence or absence of edema This might be due to the large standard deviations of the ED for cases with edema, which prevented us from observing a significant difference.
Measurement point D had the smallest standard deviation of the ED. There were significant differences in the shape and peak value of the frequency spectrum at measurement position D depending on the presence of edema. A 194 Pa difference was observed in the peak value. When an edema was present, the shape of the frequency spectrum was gentle, whereas, when edema was absent, the shape of the frequency spectrum was sharp. The frequency of the peak was approximately 3 MHz. In edema, fat cells of various particle sizes are suspended in water or tissue fluids. When propagating in a medium containing particles of various sizes, the scattering and attenuation due to their propagation result in a mild frequency response. Therefore, the shape of the frequency spectrum, which is the frequency response in the presence of an edema, is considered gentle, with no strong peaks being observed. On the contrary, in the absence of an edema, the frequency response of the input pulse remains due to the absence of scattering and attenuation media, such as fat. A peak with a center frequency of approximately 3 MHz was observed.
Based on these results, we propose that three indices can be used to understand the status of an edema. The first index is the thickness of the ED. In this experiment, the thickness of the ED was greater than 3 mm when an edema was present. The second index is the standard deviation of the ED. In this experiment, with the exception of measurement position D, the standard deviation of the ED was significantly larger in the presence of edema. The third index is the shape of the frequency spectrum. At measurement position D, the shape of the frequency spectrum differed substantially, depending on the presence or absence of an edema. For the cases with edema, the shape of the frequency spectrum was gradual. In the absence of an edema, a peak was observed in the frequency spectrum.
The presence of an edema was determined by measuring the ED. It was also determined through the sizes of the standard deviations at measurement points A, B, and C. However, this approach is inconvenient because it is necessary to perform several measurements to derive the standard deviation. At measurement point D, it was difficult to determine the presence of an edema based on standard deviation; however, it was easy to determine for its presence based on the results of the frequency analysis. At measurement points A, B, and C, it was also difficult to determine the presence of an edema based on the results of the frequency analysis. Therefore, we believe that measurement point D is desirable to easily and quickly determine the presence of an edema. The results of the frequency analysis at measurement position D can be used to easily determine the presence or absence of an edema. We believe that this method will allow us to determine not only the presence or absence of an edema, but also its degree.
CONCLUSION
In this study, we observed the ultrasonic propagation waveform on a human lower limb using a custom-made ultrasonic transducer and a pulsar receiver. We attempted to understand the state of an edema based on the information obtained from the ultrasonic propagation time waveform. We found three indices with a high possibility of understanding the state of edema. The first index was the ED or edema thickness, the second was the standard deviation of ED, and the third was the shape of the frequency spectrum of the ultrasound propagation time waveform. The results indicated that the presence of an edema could be easily and quickly determined by determining ED at measurement point D and performing frequency analyses. However, because of the small number of samples, we will further study the three indices; we believe that the degree of edema can be determined using the three indices.
ACKNOWLEDGMENTS
This work was supported in part by the Grant-in-Aid for Scientific Research B [Grant number 20H03964].
INSTITUTIONAL REVIEW BOARD PERMISSION
The study protocol was approved by the Ethics Committee of the University of Shiga Prefecture (No. 814).
CONSENT
Participants gave their written informed consent prior to study entry.
CONFLICTS OF INTEREST
The authors declare that they have no conflicts of interest.