INTRODUCTION
Type 2 diabetes mellitus (T2DM) is a complex metabolic disorder of carbohydrates, fats and proteins resulting in an inability of insulinaction and/or secretion.1 T2DM is characterized by chronic hyperglycaemia with perturbations of glucose homeostasis due to absence of regulation of postprandial glycaemia. Postprandial glyceamia is at the onset of disturbances of glucose tolerance which appears earlier before high-levels of fasting blood glucose.2 Several factors influence postprandial glycaemia: foods rich in carbohydrates, glucose digestion and absorption, insulin secretion as a result of rise of glucose levels, incretins actions and glucose intake by cells.3 Postprandial glucose is also associated to protein glycosylation to the generation of reactive oxygen species which attack DNA and membrane lipids, to increase plasma lipid.4,5 It is also a risk factor for cardiovascular diseases.6
Disturbances related to the glucose homeostasis as well as complications associated to dyslipidemia complexify management of diabetes, leading to alarming and increasing prevalence. In fact, 451 million individuals aged 18-99 years were reported to suffer from diabetes with more then 693 million of patients projected by 2045.7 Such increasing prevalence is a public health concern and constitutes economic burden for governments. It is why efforts are made everyday to improve health of diabetic patients. Several oral drugs developed are used to reduce blood sugar. These include inhibitors of digestive enzymes, which blocked alpha glucosidases (amylase and invertase), inhibitors of intestinal absorption of glucose which inhibit membrane transporters: inhibitors of Dipeptidyl peptidase-4 (DPP4) which promote insulin secretion and molecules involved in the capture of peripheral glucose.8,9 Thiazolidinediones, sylfonylureas, Metformin are oral antidiabetic drugs that play a prominent role in the treatment algorithm of T2DM.10,11 Unfortunately, despite the efficacy of those drugs side effects like hypoglycemia, nausea, vomitting, headache and constipation are still reported.12,13,14 Exploration of novel bioactive molecules from plants against diabetes has been intensified mainly with the availabilityof cell and tissue models like yeast cells and psoas mucles.14,15,16
Cameroonian pharmacopea is very rich in plants with medicinal action including Citrus sinensis, Persea americana and Allium sativum.17 They have been reported to have antihyperglycemic effects on rats in an oral glucose tolerance test.18,19 However, few studies on their action mechanisms exist to justify their efficacy. This study was designed to evaluate and compare effects of ethanolic and aqueous extracts of C. sinensis stem bark, P. americana seeds and A. sativum bulbson digestive enzymes (invertase and alpha amylase); to determine their adsorptive/glucophagic capacity; to evaluate their capacity to enhance glucose uptake by yeast cells and psoas musleas models.
MATERIALS AND METHODS
Chemicals and Reagents
Krebs solution, insulin and acarbose (GLUCOR®) was purchased in local pharmacy. Baker’s yeast, and Chronolab test kits for Glucose andamylase assays were purchased locally. Amylase Kit contained reagent R[(2-chloro-4-nitrophenyl-α-D-maltotrioside (CNPG3; 2.25 mmol/L)+sodium Chlorure (NaCl, 350 mmol/L)+calcium acetate (CH3COO)2 Ca, 6 mmol/L)+potassium Thiocyanate (KSCN, 900 mmol/L)]. Alpha amylase and invertase were purchased from Sigma Co., Louis, MO, USA.
Plant Materials
Stem bark of C. sinensis, seeds of P. americana and bulb of A. sativum were used. C. sinensis was harvested in February 2017 in Yaounde, City capital of Cameroon. A. sativum and P. americana fruits were bought at Mokolo, a local market in Yaounde, Cameroon. C. sinensis L (voucher N° 25859/SRF), P. americana mill (voucher N° 31940 HNC), A. sativum (voucher N° 44810 HNC) were identified at the National Herbarium as belonging to the families of Rutaceae, Alliaceae and Lauraceae respectively. Stem bark were isolated from C. sinensis trees. Peels of A. sativum bulbs were removed and the bulbs were isolated. Seeds of P. americana fruits were removed. Each isolated part was cut into small pieces and dried in open air (till constant weight). The dried materials were ground finely to obtain powder, from which different extracts were prepared.
Preparation of Ethanolic and Aqueous Extracts
One hundred grams (100 g) of each powder were placed in 800 mL of ethanol (95%) for maceration during 48 hours. The resulting supernatant was filtered using Whatman #1 filter paper (Whatman International Limited, Kent, England) through a funnel and concentrated to about 10% of the original volume by a rotavapor (BUCHI Rotavapor R-114, Switzerl) and at 45 °C, before complete drying in an oven at 50 °C. The same procedure was followed to prepare aqueous extract; ethanol being replaced by distilled water. After 24 h of maceration, the mixture was submitted to rotavapor. Extracts collected were stored in polyethylene bags to avoid moisture.
Assessment of Antihyperglycemic Mechanisms
Three mechanisms were evaluated: inhibition of carbohydrates digestion through the α-amylase and invertase assays, glucophagic effects through the glucose adsorption assay and stimulation of insulin-sensitivity through glucose uptake by yeast cells and muscles (psoas) assays.
Invertase inhibition Assay
Invertase activity was evaluated after hydrolysis of sucrose to form glucose. Reaction mixture was made up with 290 μL phosphate buffer, 40 μL invertase and 250 μL of sucrose was pre-incubated at 370 °C for 10 min. Then 40 μL of plant extracts at different concentrations(0.5; 1; 1.5; 2; 2.5; 5 and 10 mg/ml ) was added in all tubes except in the control containing 40 μL of phosphate buffer. After incubation at 37 °C for 15 min, all the tubes were heated 100 °C in a water bath to stop the reaction. Glucose formed in each tube was measured as described by Trinder.20 Absorbance (OD) was read at 505 nm. Another test tube containing no enzyme was used as blank for calibration. Tests were done in triplicate. Results were expressed as inhibition percentage:
((OD control-OD sample))
Inhibition (%) = ——————————————
(OD control)×100
α-Amylase Inhibition Assay
α-amylase activity was evaluated according to protocol described by Foo et al21 with modifications. Chronolab test kit was used. α-amylase (AMS) hydrolysis 2-chloro-4-nitrophenyl-α-Dmaltotrioside (CNPG3) to yield 2-chloro-4-nitrophenol (CNP),2- chloro-4-nitrophenyl-α-D-maltoside (CNPG2), maltotriose (G3) and glucose (G). The initial velocicity measuring the formation of CNP was proportional to the enzyme catalytic concentration. Absorbance was read at 405 nm. In presence of extacts at different concentration, a reduction of absorbance was interpreted as inhibition of enzyme activity. Briefly, 75 μL of reagent Rwas diluted in 6 mL of distilled water. Then , 550 mL of reagent Rwas mixed with 100 μL of extracts at 1.25; 2.5; 5; 7.5 and 10 mg/ml. Another tube containing 100 μL of distilled water served as control. All the tubes were pre-incubated for 3 min at 37 °C, and 20 μL α-amylase at (0.31 mg/mL) was introduced in the reaction mixture. All the tubes were incubated again at 37 °C for 10 min and the reaction was stopped by placing them 5 min in a water bath at 100 °C. Another test tube containing no enzyme, but 40 μL phosphate buffer served as blank. Tests were done in triplicate. Results were expressed as inhibition percentage.
((OD control-OD sample))
Inhibition (%) = —————————————
(OD control)×100
Glucose Adsorption Assay
Glucose adsorption capacity of the extract was determined according the method of Ou et al.22 Briefly, 1 ml of extracts prepared at different concentrations (5; 10; 15 and 20 mg/ml) was added to 1 mL of glucose solution of increasing concentrations (12.5, 25, 37.5 and 50 mM), the mixture was stirred well, incubated in a shaker water bath at 37 °C for 1 hr, centrifuged at 4,000 g for 20 min. The glucose content in the supernatant was determined according to the method of Trinder.20 Results were expressed as inhibition concentration. Glucose bound was calculated using the following formula:
(([Glucose]initial -[Glucose final))
Glucose adsorption (%) = ———————————————
[Glucose]initial×100
Glucose Uptake by Yeast Cell Assays
Yeasts were prepared according to the method of Cirillo.23 Commercial baker’s yeast was washed by repeated centrifugation (4,000 g for 5 minutes) in distilled water until the supernatant became clear. Then 10% (v/v) suspension was prepared in distilled water. Extracts prepared at various concentrations (5; 10; 15 and 20 mg/ ml) were added to 1 mL of glucose solution (25 mmol/L) and incubated together for 10 minutes at 37 °C. The reaction started by adding 100 μL of yeast suspension into the medium. The mixturewas vortexed and further incubated at 37 °C for 60 minutes. After 60 minutes, the tubes were centrifuged (3,000 g for 5 minutes) and glucose in the supernatant was measured according to the method of Trinder.20 A tube containing amixture without extract was used as control. Glucose uptake by yeast cells was expressed in percentage and calculated using the following formula:
((Absorbance Control-Absorbance sample))
Glucose uptake (%) = —————————————————————
(Absorbance Control)×100
Glucose Uptake in Rat Psoas Muscle Assay
Glucose uptake in rat psoas muscleof the extract was determined according to the method of Al-Awadi et al24 with modifications. Modifications included point measurements at 2h 30 min only. Psoas muscle was isolated from two anaesthetized adult rats and placed immediately in Krebs solution containing glucose (11.1 mm). Muscle tissue was cut into pieces of equal mass (0.25 g), and preincubated for 5 min in a CO2 incubator. Triple sets including muscle tissue alone (control), muscle tissue with insulin (50 mU/L), muscle tissue with both insulin and extract (5, 7.5 and 10 mg/ml) were incubated for 2.5 hrs in CO2 incubator under 95% O2 and 5% CO2 atmosphere. Changes in glucose concentrations were measured according to the method of Trinder.20 The percentage of glucose uptake was calculated using the following formula:
((Absorbance Control-Absorbance sample))
Glucose uptake (%) = —————————————————————
(Absorbance Control)×100
Statistical Analysis
All experiments were performed in triplicate. Results obtained were expressed as mean±SD from three distinct observations and as percentages. The software SPSS 20.0 (Chicago-Illinois Inc., IL, USA) was used for analyses. Chi square test were performed to compare percentages. One-way ANOVA followed by post-hoc Tukey’s test was performed to compare variable amongst groups. Using Graph Pad Prism 6.0 (GraphPad Prism INC., CA, USA), the inhibition percentages versus logarithm of the extract concentrations were plotted and inhibitory concentration 50 (IC50) weredetermined by regression. Analyses were done at 95% confidence interval.
RESULTS
Inhibition of Invertase Activity by Extracts
All the extracts inhibited invertase in a dose-dependent manner (Figure 1). Ethanolic extracts of P. americana and C. sinensis were the most efficient wtih values of IC50 of 2.69 and 2.35 mg/ml respectively, while aqueus extract of A. sativum was the most efficient of the aqueous extracts, with an IC50of 1.92 mg/ml (Table 1).
Figure 1. EEPA: Ethanolic Extract of P. americana; AEPA: Aqueous Extract of P. americana; AECS: Aqueous Extract of C. sinensis; EECS: Ethanolic Extract of C. sinensis; AEAS: Aqueous Extract of A. sativum; EEAS: Ethanolic Extract of A. sativum. Results Expressed in Percentage
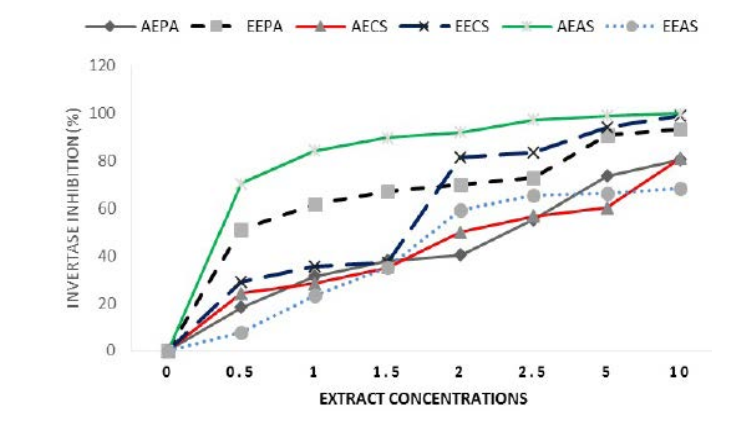
Table 1. Inhibitory Concentration 50 of Different Extracts (IC50)
Extracts |
P. americana |
Citrus sinensis |
Allium sativum |
Acarbose |
Ezymes |
Aqueous |
Ethanolic |
Aqueous |
Ethanolic |
Aqueous |
Ethanolic |
|
Invertase |
4.66
|
2.69
|
4.76
|
2.35
|
1.92
|
4.81
|
/
|
α-amylase |
0
|
6.08
|
58.09
|
0.063
|
2.85
|
0
|
2.73
|
IC50 : Inhibitory Concentration 50.; Results expressed in mg/ml of extracts |
Inhibition of α-amylase activity by extracts
All extracts partially and weakly inhibited α-amylase in a dose dependant manner except ethanolic extracts of A. sativum and aqueous extracts of P. americana that showed no inhibition (Figure 2A). Regression analysis revealed that aqueous extracts of C. sinensis was the most efficient and provided upto 91.94% inhibition of enzyme activity and an IC50=4.96 mg/ml. All other extracts had inhibitions lower than 17.06%.
Figure 2A. EEPA: Ethanolic Extract of P. americana; AECS: Aqueous Extract of C. sinensis; EECS: Ethanolic Extract of C. sinensis; AEAS: Aqueous Extract of A. sativum. Results Expressed as Percentage
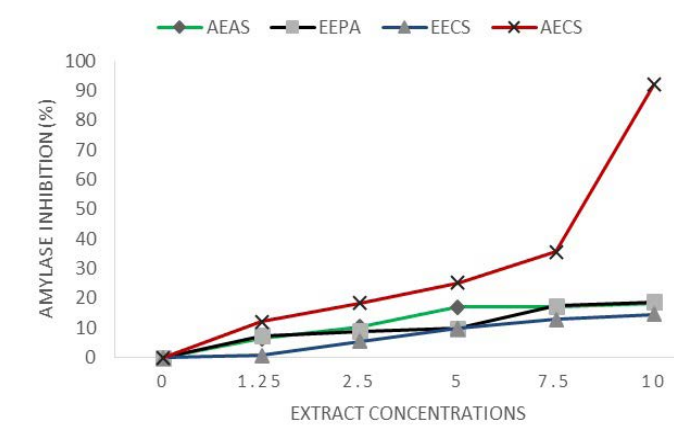
Under similar conditions acarbose used as reference drug gave an IC50 of 2.73% (Figure 2B and Table 1), higher than that of AE C.sinensis.
Figure 2B. Inhibition of alpha Amylase by Acarbose as Reference Drug
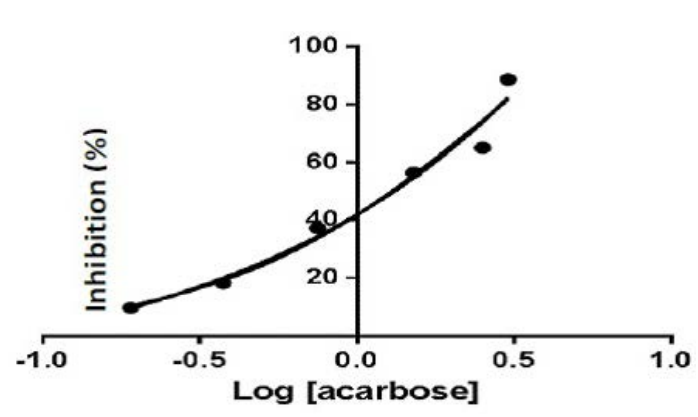
Invertase activity was reduced by extracts. IC50s vary from 1.92 (AE A. sativum) to 4.81 (EE A.sativum). Inhibition of alpha-amylase activity by C. sinensis varies from IC50=0.068 mg/ml (thanolic extracts)to 58.09 mg/ml (aqueous extract) (Table 1). Aqueous extract of P. americana and ethanolic extracts of A. sativum showed null inhibition.
Glucose Adsorption Capacity of Extracts
All extracts of different plants presented a minimum of 47.55% of glucose adsorption at the tested doses (Figure 3). Glucophagic activity observed was dose dependent. Aqueous extracts of A. sativum and C. sinensis were the most efficient with percentages varying from 75.87% to 82.68% ; 88.21% to 100% respectively, while ethanolic extractof P. americana was the most efficient with adsorption pourcentages of glucose from 86.71% to 96.35%. Aqueous extracts of C. sinensis presented the best glucophagic activity at low concentrations (<15 mg/ml).><15 mg/ml).
Figure 3. EA : Ethanolic Extract ; EE : Aqueous Extract; Results expressed as Mean±SD in Percentages *: p<0.05 when Compared to Aqueous Extracts of each Plant at the Same Concentration
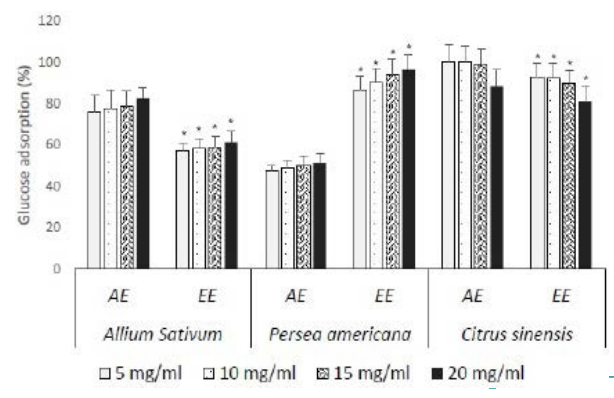
Glucose Uptake Capacity of Extracts on Yeast Cells Model
All extracts increased glucose capture by yeasts in a dose dependent manner (Figure 4). Ethanolic extracts were the most efficient with values varying from 19.14% to 45.46%, 18.24% to 54.74% and 27.56% to 37.19% respectively for A. sativum, P. americana and C. sinensis. The lowest efficacy was observed with AE of C. sinensis while EE of P. americana seeds presented the best absorption on yeast cells.
Figure 4. EA : Ethanolic Extract ; EE : Aqueous Extract; Results expressed as Mean±SD in percentages *: p<0.05 when Compared to Aqueous Extracts of each Plant at the Concentration
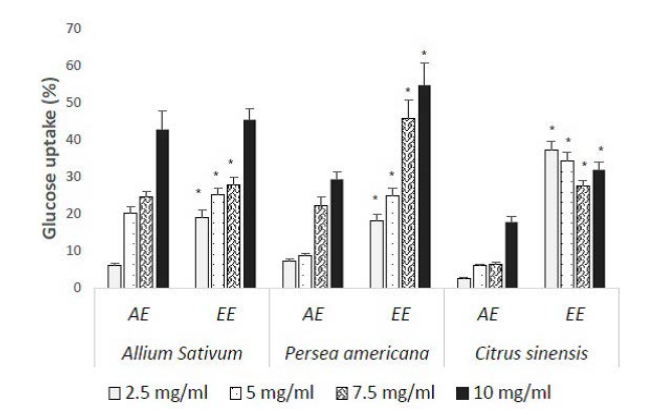
Glucose Uptake Capacity in Rat Psoas Muscle
Using insulin as reference drug, psoas tissues were capable to uptake 10.45% of glucose. It appears that all extracts were capable to enhance glucose uptake at increasing concentrations (p<0.05 vs insulin) between 14.03% to 38.56 %; above insulin performance (Figure 5). Ethanolic extracts were the most efficient with uptake varying from 24.66% to 37.66%, 34.90% to 38.56% and 18.26% to 29.29% at increasing concentrations respectively for P. americana, A. sativum and C. sinensis compared to aqueous extracts. Futhermore, increment estimated as portion of glucose uptake by muscle tissue intrinsically attributable to extracts varied from 4.21% to 27.21%; 24.45% to 28.11 % and 7.81% to 18.84 % respectively for P. americana, A. sativum and C. sinensis. Bulbs of A. sativum presented the best lowering effects on blood glucose by mediation of glucose uptake by muscle tissues.
Figure 5. EA : Ethanolic Extract ; EE : Aqueous Extract; MT: muscle tissues; Resultsexpressed as Mean±SD in percentages;*: p<0.05 when compared to insulin; a: p<0.05 when Compared to the Lowest Concentration of each Plant Extract
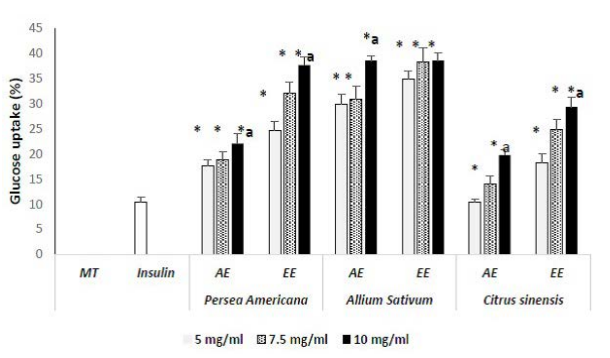
DISCUSSION
Hyperglycemia is a major symptom of type 2 diabetes and a risk factor of cardiovascular diseases. However, many bioactive molecules derived from plants like polyphenols have proved their efficacy in the treatment of metabolic disorders.25 Polyphenols are highly soluble in polar sovents like water and ethanol, reason why those two extracts were prepared from stem bark of C sinensis, bulbs of Alium sativum and seeds of Persea americana. After consumption of foods, action of digestive enzymes α-amylase and invertase in the gastrointestinal tract produces smaller molecules.15 Monosaccharrides resulting from digestion are absorbed into enterocytes to reach the blood stream, where they enter cells to supply energy and perform other biological functions.26 Treatments administred in conditions of metabolic syndrome or diabetes target interaction with one or several of the abovementioned steps. In this study, the action of different plants have been reported on digestive enzymes. Starch and saccharose are the main polysaccharides found in meals.
An evaluation of invertase activity in the presence of both extract and sucrose revealed that all the extracts inhibited invertase (Figure 1) with IC50 varying from 1.92 (AE A. sativum) to 4.81 (EE A.sativum) (Table 1). Sucrase or invertase is a bifunction-al enzyme also called sucrase-isomaltase which hydrolysis sucrose and isomaltose substrates.15 If aqueous extract of P. americana and ethanolic extracts of A. sativum showed no inhibition (null) of alpha amylase at the tested concentrations (Figure 2A), EE of Citrus sinensis inhibited α-amylase instead with an IC50=0.063 mg/ml (vs 2.73 with acarbose) (Figure 2B and Table 1). α-amylase hydrolyses the alpha bond linked
polysaccharides of starch producing di- and mono-saccharides. Partial inhibition of the enzyme by C. sinensis extracts reduces its activity and prevents formation of monosaccharides.12,13,14,15 Inhibition of amylase and invertase by extracts delays absorption of consumed carbohydrates and reduces plasma glucose.12
All the extracts strongly inhibited invertase (Figure 1), but only the AE of P. americana and EE extract failed to inhibit the two enzymes. Inhibitors of those enzymes surely posssess in their structures functions similar to their substrates starch and sucrose although plant extracts do not possess such complex structures in their contents. Acarbose used as reference drug under the same experimental conditions provided an IC50 of 2.73 mg/mL, higher than C. sinensis extracts. Acarbose has a structure similar to oligosaccharides and therefore inhibits α-glucosidases in a dose dependent manner, preventing the formation of glucose.27 Flavonoids and alkaloids present in Allium sativum and americanaextracts can justify their efficiency as well.18,19 In fact, myricetin, quercetin, kaempferolare inhibitors of α-amylase and α-glucosidase while alkaloids like berberin are reported to be able to bind to α-glucosidases and prevent sucrose fixation.28,29,30,31 Their use to inhibit carbohydrates digestion is therefore beneficial in the management of diabetes because absorption of glucose is reduced and and postprandial hyperglycemia obviously.32,33
The residual glucose resulting from digestion can be trapped in the intestine limiting its absorption by adsorption, reducing its availibility and diffusion into blood; reducing glucotoxicity as well. Adsorptive capacity through glucophagic activity of extracts revealed high percentages of glucose adsorption (Figure 3). Flavonoids and fibers content of extracts can justify such results. Insoluble fibers are capable of binding to glucose reducing its availibility and therefore its intestinal absorption.34 Also, they can reduce viscosity of secretions in intestial lumen slowing or preventing diffusion. Absorbed glucose present in the blood can condense with hydroxyl groups of flavonoids to form complex molecules like glycosyl-flavonoids.35 Decrease in glucose concentration contributes to reduce harmful effects of high plasma glucose on hemoglobin and other proteins, preventing glycation and complications such as retinopathy, neuropathy, and nephropathy observed in diabetic patients.36,37,38
Finally, the extracts were tested for their absorption capacity at the cellular levels. Glucose transport mechanism through cell membranes using yeast cells and psoas culture as models have been of great interest in the understanding of physiological processes of hypoglycemic activities of extracts.39,40 Results revealed that all the extracts stimulted glucose entry in yeast cells (Figure 4). In fact, extracts are capable of stimulating glucose membrane transporters, just like many oral antidiabetics drugs.39,40 On yeast membrane, glucose is transported via specialised stereospecific groups of tranporters called hexose transporters (HXT1 to HXT17) and specific molecules Snf3 and Rgt2, present on the membrane and involved in its capture. Binding of glucose to Snf3/Rgt2 located on yeast cell membrane permits phosphorylation of two co-receptors Mthl and Std 1 of Rgt1 (transcriptional repressor in charge of down regulation of the HXT gene) exposing Rgt1 to phosphorylation by a protein kinase A, causing glucose to diffusion accross the membrane.41,42
Effectiveness of glucose absorption on yeast membrane mediated by extracts was confirmed by muscle cells tested ex-vivo, on extracts capacity to boost insulin action (Figure 5). Glucose absorption by cells is very important in glusose homeostasis. Upto 80% of glucose fixation depend on insulin action.43 This study revealed that psoas in the presence of insulin produced 10.45% of glucose uptake. Insulin in the presence of each plant extract produced values higher than 10.45% in a dose dependent manner (p<0.05). Similar observation was done with A. aspera extract.44 Ability of extracts to boost insulin action can be attributed to polyhenols and alkaloids content of plants which act through stimultion of GLUT4 and/or AMPK activation or insulin secretory action.45 Polyhenols stimulate glucose capture by muscle via activation of AMPK by mechanisms similar to thiazolinediones.46,47
The enhanced action of extracts alongside with insulin on rat psoas muscles has been reported with maltitol.48 Alkaloids like berber in stimulates direct glucose absorption mediated by AMPK without involvement of GLUT4.49 Ability of extracts mainly ethanolic extracts to boost insulin action can be attributed to polyphenols known to stimulate binding of insulin to IRS receptors, to increase the number of insulin receptors or/and to increase insulin sensitivity.44,45,46,47,48,49,50 Enhanced uptake of glucose by cells via joint action of insulin and extracts may stimulate glucose clearance from blood, through improved insulin resistance. Enhanced uptake of glucose also promote post absorptive utilisation in glycolysis for energy supply.44 Absorption values above 10.45% and increments due to extracts varying from 4.21 to 28.11 % respectively for P. americana and A. sativum (Figure 5) cannot be intrinsically attributed to the stimulation of insulin action, because in vitro, extracts could adsorb part of glucose before insulin action, lowering its concentration in the medium. This study however demonstrated that, the cumulative actions of extracts on enzyme digestion, glucose adsorption and insulin action lead to reduced hyperglycemia, targeted in the first treatment outcome in type 2 diabetes. Reduced hyperglycemia also limits progression to complications.
LIMITATIONS OF THE STUDY
Limitation of this study includes measurement of glucose concentration at 2 h 30 min only instead of different time intervals for better follow-up of absorption. Also, no reference drugs were used in invertase and glucose adsorption assays. Their use will have permitted better appreciate of the extracts’ efficacy.
CONCLUSION
Extracts from bulbs of A. sativum, seeds of P. americana and stem bark of C. sinensis exert their antihyperglycemic effects via inhibition of carbohydrate digestive enzymes, adsorption of glucose and stimulation of glucose absorption by cells. Stem bark extracts of C. sinensis, mainly ethanolic extracts are more effective on inhibition of digestion and glucose adsorption while bulbs of A sativums timulates glucose absorption in cells than other plant extracts. Bulbs of A. sativum, seeds of P. americana and stem bark of C. sinensis based on their efficiency and action mechanisms act like oral diabetic drugs. Therefore their use should be encouraged in the management of metabolic disorders.
ACKNOWLEDGEMENTS
Not applicable.
ETHICAL ISSUE
Not applicable.
CONFLICT OF INTEREST
The authors declare that they have no conflicts of interest.