INTRODUCTION
Approximately 300 children are diagnosed each year in the United States with retinoblastoma (Rb), the most common intraocular cancer among childhood malignancies.1,2 The severity of the disease depends primarily on the size and location of the primary tumor. If dissemination occurs Rb can spread throughout the retinal to the vitreous region, central regions of the brain, to the bones and bone marrow. Metastases significantly reduce the survival rate. Treatments can include enucleation, systemic or local chemotherapy, focal therapy, radiation therapy and in rare instances stem cell transplantation.3 These treatments emphasize on the child’s life first, while vision and preservation of the eye are of secondary concern.4
Disruption of the blood-retinal barrier (BRB), leaky vessels and high-levels of angiogenic responses including vascular endothelial growth factor (VEGF) and the active platelet-derived growth factor receptor-beta (PDGFR-β) signaling, are characteristics of Rb.5-7 Therefore, targeting the tumor and the angiogenic response may provide an alternative therapeutic strategy to this disease. In the last decade, Rb research has shifted to focus more on identifying and successfully targeting new pathways that are: 1) tumoricidal, 2) safer for healthy cells necessary for homeostasis and, 3) capable of being administered locally. In our pursuit to develop novel Rb therapeutic strategies we recently identified the PDGFR-β as being highly active in Rb disease.5 We targeted the PDGF-PDGFR-β signaling pathway using imatinib mesylate (IM, akaGleevec® from Novartis), a protein-tyrosine kinase inhibitor approved to treat acute lymphoblastic leukemia (ALL) in adults and children that are Philadelphia chromosome (summarized in)8 positive, among other malignancies, and showed in vitro reduction of Rb growth, invasion, and survival in an AKT-, MDM2-, and NF-ĸB-dependent manner.
Current therapies against Rb act on both the tumor cells and the healthy, non-malignant, cells within the stroma. We previously demonstrated melphalan-induced upregulation of ICAM-1 and apoptosis in retinal microvascular endothelial cells.9,10 Apoptosis of these cells release pro-inflammatory cytokines and chemokines to the microenvironment. In this study, we investigated in vitro the effects of disruption of the PDGF-PDGFR-β signaling pathway on primary human retinal microvascular endothelial cells (hRECs) cellular proliferation and function prior to transitioning into pre-clinical assessment of the PDGF-PDGFR-β signaling pathway in Rb. Our work demonstrated that disruption of this signaling pathway is limited to reducing Rb survival without affecting hRECs viability and organization. This work supports our hypothesis that anti-PDGFR-β therapy could be a tumor-specific therapy for use in children afflicted with Rb.
MATERIALS AND METHODS
Cell Lines and Cell Culture Conditions
Primary human retinal microvascular endothelial cells (hRECs, ACBRI 181) were purchased from Applied Cell Biology Research Institute (Cell Systems, Kirkland, WA, USA). Cells were cultured using four different conditions: untreated, recombinant human Platelet-Derived Growth Factor (rhPDGF, 10 ng/mL), Imatinib Mesylate (IM, 10 μM), and the combination of rhPDGF+IM. Y79 (ATCC, HTB-18) Rb cells11 were purchased from American Type Culture Collection (ATCC) (Manasas, VA, USA) and cultured as described above.
Cell Proliferation Assay
Cell proliferation studies were performed using the CellTiter 96® AQueous One Solution Cell Proliferation Assay (Promega, Madison, Wisconsin, USA) as before.12 Briefly, 1.0×104 UM cells per well were cultured in the conditions described above. CellTiter reagent was added at a concentration of 20 μL per 100 μL volume per well at specific time points of 0-, 48-, 72-, and 96 hrs after culture. Cells were incubated at 37 °C for 2 hrs before absorbance was read at 490 nm using a 96-well plate reader. Values expressed as mean±SEM, n=3 with 4 replicates. Statistical analysis done using Prism Graph Pad.
qPCR Analysis
RNA from 1.0×106 hRECs was extracted following the QiagenmiRNeasy Mini Kit (Qiagen, Valencia, CA, USA) manufacturer’s recommendations. Synthesis of cDNA was performed using the SuperScript® VILO™ (complementary deoxyribonucleic acid) cDNA Synthesis Kit (Life Technologies, Grand Island, NY, USA). Following manufacturer’s directions, we used 100 ng of ribonucleic acid (RNA) combined with the Reaction Buffer and the Enzyme Mix. We used the following Human TaqMan® Gene Expression Assays: HPRT1 (Hs02800695_m1), PDGFRB, and FLT1, all from Life Technologies (Grand Island, NY, USA). A final volume of 10 μL was loaded into each well after combination of TaqMan® Universal Master Mix, cDNA, primers and Nuclease Free water. Plates were run using Roche® Light Cycler 480 and data were analyzed using the Comparative ∆CT Method as before.12,13 Values expressed as mean±SEM, n=3 with 4 replicates.
Tube Formation Assay
hRECs were cultured on Reduced Growth-Factor Matrigel (BD Biosciences, Bedford, MA, USA) in complete media (10% FBS, Cell BioSystems) or low serum (0.2% FBS). Additional culture conditions included rhPDGF and IM. Images were taken at both 6- and 18 hrs, respectively using a Nikon C1 confocal microscope using 4x objective. Results are representative of two independent experiments; three fields were taken per group at both 10x (data not shown) and 4x.
Flow Cytometry Analyses
Monocultures of Y79 Rb, hRECs and co-cultures of Y79 Rb and hRECs (Rb:hREC) were treated using the conditions described above. Cell cultures were harvested at 24 hrs, fixed in PBS/2% paraformaldehyde, and labeled with anti-human PDGFR-β APC or with anti-VEGF-A AF700 (BioLegend, San Diego, CA, USA). Data acquisition done using a Bio-Rad ZE5 Cell Analyzer (aka YETI, Propel Labs, Fort Collins, CO, USA); analysis done using FlowJo vX.0.5 (Tree Star). Each analysis done on a minimum of 50,000 events.
RESULTS
Cell Proliferation of Human Retinal Endothelial Cells is Independent of PDGF-PDGFR-β signaling
We first measured Y79 proliferation using the tyrosine kinase inhibitor (TKI) imatinib mesylate (IM), a known inhibitor of PDGFR. We had previously identified 10 μM as the most efficacious concentration.5 Inhibiting the PDGFR-β signaling with IM significantly reducedthe proliferation of Y79 Rb cells at 72 hrs (Figure 1A). As a physiological control, we stimulated Y79 Rb cells with recombinant human PDGF-BB (rhPDGF), which we discovered to be highly abundant in the tumor microenvironment of Rb.5 To investigate the role PDGF-BB may have on hRECs and to test if disrupting the PDGF-PDGFR-β signaling pathway by IM may be toxic to hRECs, we cultured hRECs in the same conditions as Y79 Rb cells. We tested proliferation over 96 hrs using MTS cellular proliferation assay and measured no changes in cellular proliferation (Figure 1B). These results illustrate how hREC proliferation occurs independent of PDGF-PDGFR-βsignaling. Next, we investigated the expression levels of PDGFRB to measure receptor activity. We harvested mRNA from hRECs and Y79 cells to measure the expression of FLT1 (VEGFR1) and PDGFRB relative to HPRT1, an endogenous control. The mRNA expression of FLT1 was higher in hRECs compared to Y79 Rb cells. However, PDGFRB mRNA expression was not detected (Figure 1C) in hRECs, while high expression was uncovered in Y79 Rb controls. Taken together, these results demonstrate how targeting of PDGF-PDGFR-β signaling will not have anti-proliferative effects in hRECs, as they do not express PDGFRB.
Figure 1. hREC Proliferation Occurs Independent of PDGF-PDGFRb Signaling
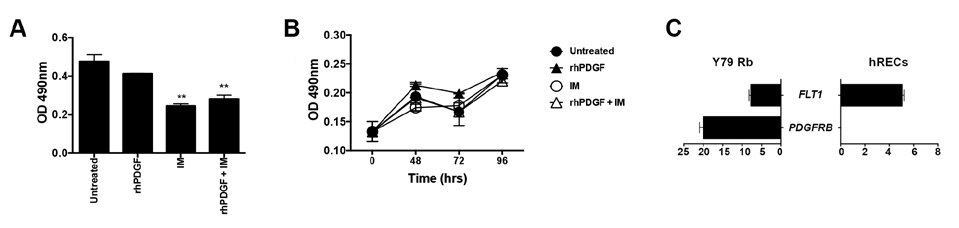
Disruption of the PDGF-PDGFR-β signaling Pathway Does not
Affect Angiogenic Activity in hRECs
To test the hypothesis that anti-PDGFR-β treatment will not affect the normal, non-pathological, angiogenic activity of hRECs, we cultured hRECs on reduced-growth factor extracellular matrices under the following conditions: complete media (with 10% FBS), low serum (0.2% FBS), and low serum+rhPDGF (10 ng/ mL). Non-pathogenic organization of hRECs display complex tubular structures similar to blood vessels (Figure 2A, left).Under stressful conditions, simulated through serum starvation, hRECs do not readily organize into these branched structures, as shown in Figure 2A, middle. Next, we tested if the PDGF-PDGFR-β signaling could restore hRECs ability of tube formation. Results in Figure 2A, right demonstrate this signaling pathway cannot restore hRECs angiogenic activity in vitro, defined by the tube formation assay.
Figure 2. PDGF-PDGFR-β signaling is Unable to Initiate Tube Formation

As a next step, we tested if the PDGF-PDGFR-β signaling pathway is capable of promoting or stimulating tube formation. hRECs were all cultured in complete media and under the following conditions: untreated, stimulated with rhPDGF (10 ng/ mL), IM (10 μM), and rhPDGF+IM. We found no morphological changes in hREC vascular organization (Figure 2B) across the different cell culture conditions. Ultimately, these results indicate hRECs angiogenic function is independent of PDGF-PDGFR-β signaling.
PDGFR-β Contributes to hREC VEGF-A Production
To evaluate cellular interactions and potential role(s) PDGFPDGFR-β signaling may play in the Rb tumor microenvironment, we set up a co-culture system composed of Y79 Rb cells and hRECs using the same conditions described above. Y79 Rb cells were labeled with the non-toxic dye carboxyfluorescein succinimidyl ester (CFSE) to trace the cells during single cell analyses, while leaving the hRECs unlabeled (Figure 3A). Co-cultures of Rb:hRECs were done using two different ratios, 1:1 and 1:10, to examine potential cell number-dependent roles. Flow cytometry analysis for the evaluation of the percentage of PDGFR-β+ cells showed differences between Y79 Rb cells and hRECs. About half of the Y79 Rb cells display immune positivity for PDGFR-β, while a minimal percentage for hRECs (Figure 3B, top left), confirming our mRNA results in Figure 1. In our co-cultures, we measured reductions in the percentage of cells expressing PDGFR-β after IM treatment, as shown in Figure 3B, top right.
Figure 3. Treatment with IM Reduces hREC VEGF Production
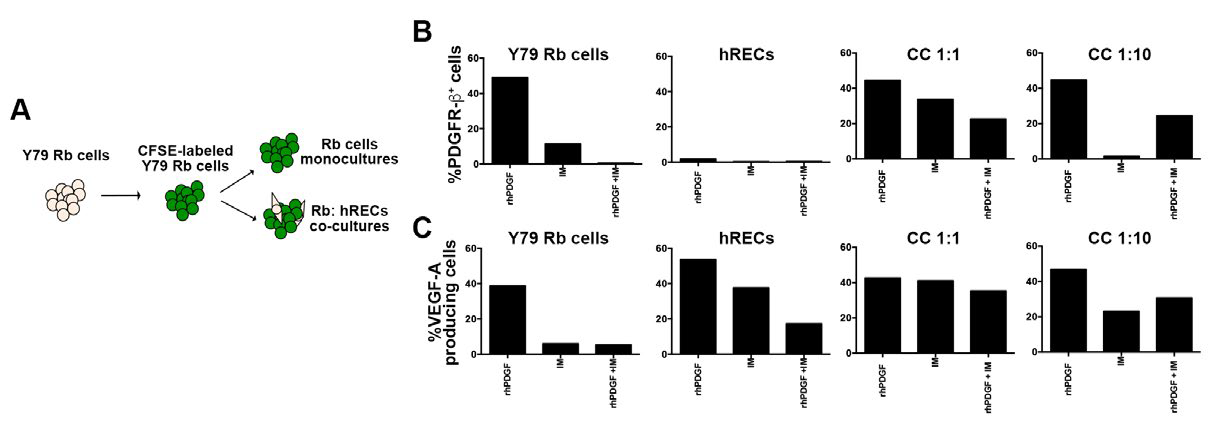
Next, we examined the percentage of VEGF-A+ cells in Rb cells and hRECs after disruption of the PDGF-PDGFR-β. There was a reduction in the percentage of vascular endothelial growth factor-A (VEGF-A)-producing cells in both mono- and co-cultures after disruption of the PDGF-PDGFR-β signaling pathway (Figure 3C). Collectively, our results show a reduction in Y79 Rb cells expressing PDGFR-β+ and a reduction in VEGF-Aproducing cells in both Rb tumor cells and hRECs.
DISCUSSION AND CONCLUSION
Retinoblastoma (Rb) remains the most common intraocular malignancy afflicting children. While survival rates are typically above 95% in the United States, clinical management is challenging in developing countries. New mechanisms for drug delivery have been developed in recent decades, specifically those of super-selective intra-ophthalmic artery chemotherapy (SSIOAC) and intravitreal chemotherapy (IVT).14,15 These new treatment mechanisms have garnered much attention into increasing ocular salvage. Still, they often have severe side effects for the pediatric population including ischemia, neutropenia, a higher risk for development of a secondary malignancy, and blindness.14,16-18
In recent years emerging efforts at the development of novel therapeutics for the control of Rb tumor cells, while being safe for non-malignant cells for homeostasis, have increased. Therapeutic interventions at controlling VEGF have not succeed, suggesting other factors in addition to VEGF play pivotal roles in Rb. We recently identified the PDGFβ-PDGFR-β signaling pathway as an active pathway in Rb. Our work demonstrated a novel-signaling pathway for potential targeted therapy that could improve ocular survival in advanced Rb. While our initial studies focused on targeting of the tumor cells, here, we investigated the effects of pharmacological disruption of this pathway in microvascular retinal endothelial cells, key players in the blood-retinal barrierhighly affected by many ocular diseases including Rb and diabetes retinopathy. All of these as necessary steps prior to transitioning into pre-clinical evaluation.
Previous work from our team9,10 identified how melpha lan and carboplatin, two commonly administered chemotherapies to treat Rb, increase retinal endothelial cell death and inflammation.9 A recent report discovered superselective intraophthalmic artery chemotherapy (SSIOAC) administration, while allowing for higher concentrations of localized drug delivery, does affect the contralateral eye with concentrations of drug in plasma being similar to those of systemic (or intravenous) administration.19 As endothelial cells are necessary to supply the healthy neural retina with nutrients via blood supply, significant loss of these cells can result in neurodegeneration and, ultimately, vision loss. These endothelial cells are also the primary component of the BRB that prevents cellular infiltration into the healthy retina and use of an antiPDGFR-β therapy could help maintain this essential structure.20,21 Our study demonstrates the pro-apoptotic effects of IM are Rb cell specific, as hRECs proliferation is not affected. In contrast to human umbilical vein endothelial cells (HUVEC), which are widely used in in vitro studies of endothelial cells, hRECs cannot signal through the PDGFR-β. This was demonstrated in our studies by genomic and flow cytometry analyses. Of note, we measured a reduction in the %VEGF-A+cells in the hRECs and Y79 monocultures. Work from Pennock and colleagues22 suggested VEGF-A could signal through the PDGFR-β to promote cell viability during hypoxic conditions. Studies in our laboratory showed no difference in VEGFA, VEGFR mRNA in Y79Rb cells (data not shown) when the PDGFβ-PDGFR-β signaling pathway is disrupted. Collectively, this work suggests anti-PDGFR-β therapy could be an Rb tumor-specific therapy.
The present study investigated the role of the PDGFPDGFR-β signaling pathway in hRECs. As a functional readout of disruption of this signaling pathway we used a genomic (PDGFRB mRNA expression), flow cytometry (percentage of PDGFR-β+ cells and VEGFA-producing cells), and angiogenic (tube formation assay) approaches. We are cognizant of the limitations of the study as we focused on a small cohort of hRECs properties in vitro. We utilized the primary hRECs, which are isolated after medical enucleations to link our cellular results to the translation of human disease. These are considered “truly microvascular cells from the retina”,23 in contrast to macrovascular cells from human umbilical vein or human artery. Literature reports demonstrated the presence of PDGFR-β in human umbilical vein endothelial cells (HUVEC), which contrast to the results presented in this work using hRECs, highlighting the need to use the appropriate models to translate the work. Further studies aim at investigating endothelial cell leukostasis and toxicity in vivo using a Rb xenograft model developed by our colleagues.24 Histopathological findings will address ocular effects after in vivo disruption of the PDGF-PDGFR-β signaling pathway and will allow for more in-depth analyses at cellular interactions within the microenvironment.
Children with Rb face cosmetic and psychological challenges if undergo enucleation. Those who undergo systemic chemotherapy, although there is tumor reduction, become more likely to develop other cancers. Multiple adverse effects are noted in those who receive intra-arterial delivery of the chemotherapeutics. Radiation therapy, similar to chemotherapy, has its adverse effects and lacks the ability to distinguish between healthy and cancerous cells. Targeted therapy has the potential to deliver more effective therapy, as it is designed to act on specific molecular targets, such as PDGFR-β, in a cell-specific fashion.
ACKNOWLEDGEMENTS
We would like to thank the Flow Cytometry and Cell Sorting Core facility, the Molecular Resource Center, and the members of the Morales-Tirado Laboratory. This work was funded by the Neuroscience Institute (ZKG), the Gerwin Fellowship (ZKG, VMT), St. Baldrick’s Foundation (ZKG), Fight for Sight (ZKG), SJCRH Chair Endowment (MWW), The Ocular Oncology Fund (VMT, MWW), 930 Friends (VMT), and Research to Prevent Blindness (PI: JCF).
AUTHOR’S CONTRIBUTIONS
Conception and design of the work: MWW, and VMT; data acquisition, analysis, and interpretation: ZKG, ASI, MWW, and VMT; manuscript draft and significant contribution in intellectual content: ZKG, ASI, MWW, and VMT. All authors approved final version of manuscript. The following authors have no competing interests: ZKG, ASI, MWW, and VMT.
DATA AVAILABILITY STATEMENT
The chemical structure and compound summary of imatinibmesylate are available in PubChem, https://pubchem.ncbi.nlm.nih. gov CIDs: 123596.
All cell lines utilized in this investigation are commercially available: Primary human retinal microvascular endothelial cells are available from Cell Systems, https://cell-systems.com Cat. # ACBRI 181; Y79 Rb cells are available at the American Type Culture Collection (ATCC), www.atcc.org Cat. # HTB-18.
ETHICS APPROVAL AND CONSENT
This study did not require approval by The University of Tennessee Health Science Center Institutional Animal Care and Utilization Committee (IACUC) and Institutional Review Board (IRB).
CONFLICTS OF INTEREST
The authors declare that they have no conflicts of interest.