INTRODUCTION
Venomous snakebites are a common health problem in most tropical and sub-tropical areas. Some snake species can be fatal or induce permanent disabilities, resulting in economic losses and reducing the quality of life. Particularly in Latin America is possible to find several venomous snake genera, for instance, Bothrops, Micrurus, Lachesis, and Crotalus, mainly related to small rural villages, often with deficient health care systems. Some estimations have calculated the global incidence of snakebite (lower estimation) by 100.000 inhabitants in 6.28, being 19.04 for the area of Venezuela and Colombia.1 The mortality rate in the Americas region was proposed 0, 05 for (by 100.000 inhabitants) Venezuela (up to 40-year-old patients) and 0, 04 for the region, a rate considered moderate in comparison with data from Africa or Asia2 Although snakebite does not have an epidemic potential of infectious and vector-borne parasitic diseases, it is important to emphasize that the yearly mortality caused by snakebite is much greater than that attributed to several presently recognized neglected tropical diseases in the Americas, including dengue hemorrhagic fever, cholera, leishmaniasis, schistosomiasis and Chagas’ disease,3 making necessary more investing in research related to antivenom production and pathophysiology of snakebite.
Crotalus envenomation is characterized by neurologic, myotoxic, and nephrotoxic syndromes, which are present in a variable intensity, depending on the amount of venom inoculated victim weight, and snake size.4 In Venezuela, the most common species is Crotalus durisus cumanensis, which is found in the driest areas of the country. Additionally, in Venezuela have been reported various crotalid endemic species, such as Crotalus vegrandis5 endemic to Uracoa, Monagas state, and Crotalus pifanorum, 6 endemic for Guarico state, which are placed in areas where may cause snakebites to humans and domestic animals.
Some non-conventional effects have been reported for Crotalus venoms. Cardiotoxicity has been demonstrated in experimental animals inoculated with Crotalus durissus cumanensis venom.7 Other authors have reported cardiotoxicity in horses, bitten by rattlesnakes.8 Among cardiotoxicity, several alterations counting atrial fibrillation9 and pro-arrhythmogenic activity of a protein isolated from Crotalus durissus collilineatus venom10 have been described. Beyond the relative scarcity of information about cardiac disturbances produced by snake venoms; to the best of our knowledge, the reports about the pathophysiology of snakebite of C. vegrandis and C. pifanorum are very scarce.
The current work aimed to characterize the pro-arrhythmogenic activity of C. vegrandis and C. pifanorum venoms and the proficiency of commercial antiserum for inducing cardiotoxicity reversion.
MATERIALS AND METHODS
Reagents
Polyvalent antiophidic serum was purchased from Biotecfar C.A (Pharmacy Faculty of the Universidad Central de Venezuela, Caracas, Venezuela).
Venom Collection
Venom was collected for adult snakes’ specimens of C. vegrandis and C. pifanorum located at the snake collection at “Parque del Este” (Caracas, Venezuela) and the Toxicology Unit of “Lisandro Alvarado University”, Veterinary Sciences Faculty (Barquisimeto, Venezuela). The venom was extracted by trained personnel. Venom from the different snakes was pooled, lyophilized, and stored at -70 °C until use.
Mice
Six- to eight-week-old male BALB/c mice, housed in Plexiglas cages, with water and commercial food ad libitum and 12 h dark/ light cycles. Experiments in Venezuela were performed in strict accordance with the Bioethics and Biosafety Norms (3rd edition) and approved by the Fondo Nacional de Ciencia y Tecnología de Venezuela (FONACIT), the Ministerio de Ciencia y Tecnología of Venezuela (2011), the Asociación Venezolana para la Ciencia de los Animales de Laboratorio, and the International Ethical Standards for Research Biomedical in Animals of the World Health Organization (WHO) (1982); animal handling and experimental procedures were approved by the Comité de Bioética Institucional of the Medical Faculty, Lisandro Alvarado Unuversity, Barquisimeto, Venezuela.
Electrocardiographic Recordings and Venom Inoculation
Electrocardiography (ECG) was performed using a bipolar system in which the electrodes were placed subcutaneously at the xiphoid cartilage (positive electrode), right shoulder (negative), and left shoulder as previously described.11 Electrodes were connected to a Bioamp amplifier (AD Instruments, Bella Vista, Australia) and ECG recordings were digitalized through a PowerLab 8 sp A/D converter (AD Instruments, USA). Digital data was analyzed with Chart software for Windows v7.3.1 (AD Instruments, USA), with events registered to 1 K/s and filtered to 60 Hz. Continuous ECG records were obtained for determining basal heart rate, defined as the point where there was no variation above 5%.11 At this point, NMRI albino mice were divided into five groups: 1) inoculated with 8 mg/Kg of crude venom of C. vegrandis (CV, n=6); 2) inoculated with 8 mg/Kg of crude venom of C. pifanorum (CP, n=7); 3) inoculated with 8 mg/Kg of a mixture of crude venom of C. vegrandis with a commercial polyvalent antiserum (Biotecfar C.A) (CV-AV, n=5), 4) inoculated with 8 mg/Kg of a mixture of crude venom of C. pifanorum with a commercial polyvalent antiserum and (CP-AV, N=4) 5) 8 mg/Kg of commercial polyvalent antiserum (AV, n=5). Animals with visible behavior of discomfort (behavior compatible with pain as arching the back, piloerection or aggressiveness), respiratory distress, or agonic ECG pattern were considered a final point and sacrificed for ethical reasons.
Previous to injection, the mixture AV-Venom was pre-heated at 37° for 30-minutes to achieve the inactivation of snake venom. The mixture (venom/antivenom) was performed as indicated by the supplier for treatment of snakebite, considering one vial of antivenom for neutralizing 20 mg of venom (both species). The electrocardiograms were registered continuously each 1 min, until 6-hours post-injection.
Tissue Sampling and Histological Processing
Immediately after electrocardiographic analysis, mice were sacrificed by barbituric overdose (40 mg/Kg) and hearts were collected from mice and placed in 10% neutral buffered formalin for 24 h at room temperature, followed by incubation in 70% ethanol until processing. Heart samples were then cut in a coronal shape and embedded in paraffin (Tissue Embedding Station Leica EG1150 H), and 3 μm tissue sections were prepared (Microtome Leica 2125 RTS), dewaxed and rehydrated, stained with hematoxylin/eosin (H&E) staining and mounted permanently in EukittÂs quick-hardening mounting medium (Biochemika, Fluka analytical). Sections were analyzed on a Leica microscope (Leica DM50) using the 20- 40x magnification objectives.
Creatine Kinase Muscle and Brain Activity Measurements
The cardiac isoform of creatinine kinase (creatine kinase muscle and brain (CK-MB)) is an intracellular enzyme used as a marker of cardiac cell disruption in models of cardiac necrosis.12The enzymatic activity of CK-MB was determined following the manufacturers instructions (Elitech®). Briefly, 10 µL of serum was taken by cardiac puncture and stored at -20 °C until use was plated on a 96 well-plate and mixed with 250 µL of work reagent provided by the manufacturer. Absorbance at 350 nm was determined at 37 °C by a Tecan absorbance reader, with measurements performed every 60-seconds. CK-MB activity was calculated with the mean of differential among the readings and multiplying the result by 8,254, a constant value estimated, taking into account reference values of CK-MB activity. Intraassay variation was expected to be 25%.
Statistical Analysis
For in vivo experiments, data are shown as means±SEM. Significance was evaluated by Student’s t-test when two groups were compared, by One-way analysis of variance (ANOVA) followed by the Tukey or the trend to right post-test for the analysis of three groups using GraphPad Prism 5.00 software (La Jolla, CA, USA).
A multivariate ANOVA was performed to individually compare the electrocardiographic (ECG) parameters studied. A linear discriminant analysis (LDA) was applied to observe in which proportion the levels of cytokines were able to discriminate the study groups, as was published in our previous research.13 LDA relates a variable measured in nominal scale (dependent) with a group of continuous variables, reducing dimensionality while preserving as much of the class discriminatory information as possible. The model assigns to each variable an axis, from classical X, Y, and Z to transformed space. Space reduction is based on correlation tests, where the model chooses the less correlated variable (s), which contributes to group discrimination.13 Thus, with this method method we can determine the most contributing variables, and enables the prediction of the adscription of each individual to the different groups studied with a certain probability. For this analysis the following ECG parameters were: (RR) interval, Heart rate, QTc, QRS length, Tpeak Tend Interval (s), R, S, and T amplitude, and ST height.
Finally, a χ2 analysis was performed for a semi-quantitative comparison of all types of arrhythmias. For this analysis were considered the following qualitative ECG disturbances were: ectopic beats (ventricular and supraventricular), 2nd or 3rd degree AV blockage, and repolarization disorders. Each trace was considered as “normal” (none qualitative alteration) or abnormal (at least one qualitative alteration) and registered in a double-entry table for statistical analysis.
RESULTS
For the study of cardiotoxicity of C. vegrandis and C. pifanorum venoms, we analyze structural and biochemical patterns that could be suggestive of cardiac damage, compared with normal controls. Histopathological studies (Figure 1A). Only the CV group showed moderate changes suggestive of cardiac toxicity, as cardiac fiber tumefaction and vacuolization, and these changes were not observed in the CV-AV group. No alterations in heart histology were observed in the CP group. Unexpectedly, measurement of the cardiac isoform creatine kinase (CK-MB) offered a slightly different picture, with a significant and moderate elevation of serum enzymatic activity in all groups treated with Antivenom (Figure 1B).
Figure 1. Morphological and Biochemical Assessment of Cardiac Damage
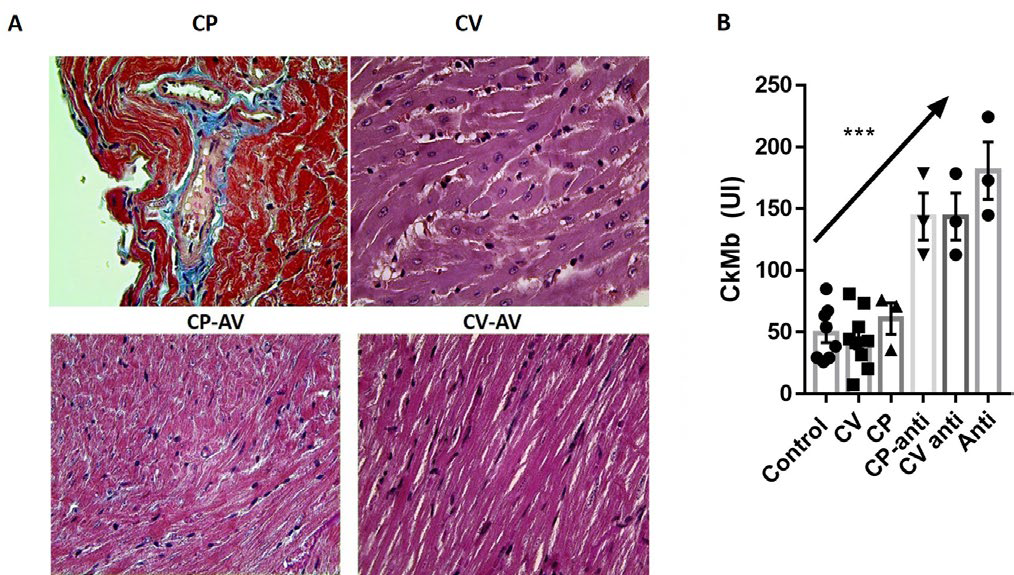
Next, the quantitative analysis of ECG parameters was performed, for both venom and antivenom groups relating to normal controls. Globally, C. vegrandis (CV) venom was able to induce a significant flattening of S and T waves (Figure 2A), and it was reverted when the CV was incubated with the commercial antiserum (anti-venom (AV)). CV induced delay of conduction from auricles to the ventricle, reflected in a significant increase of PR interval. Unexpectedly, the QT interval was significantly enlarged in animals treated with neutralized CV venom.
Figure 2. Electrocardiographic Parameters Comparison
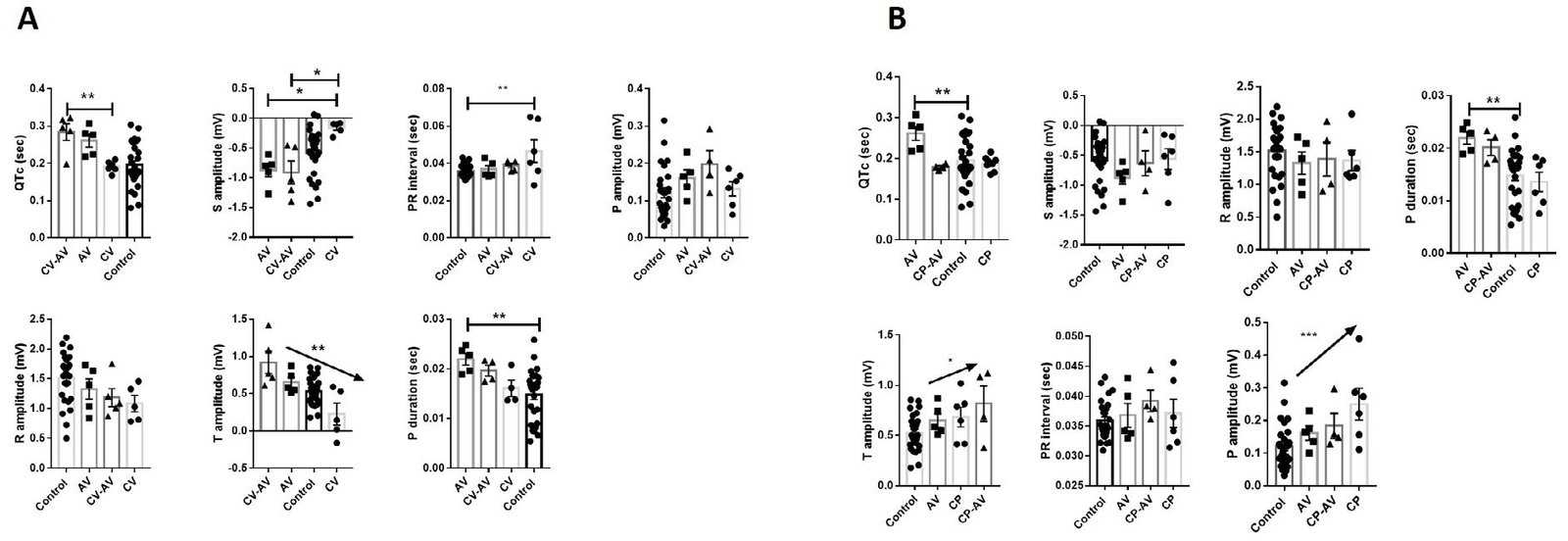
For CV venom comparison (Figure 2B), it seems that Crotalus pifanorum (CP) or CP-AV showed lesser significant changes than observed for the CV group, except for a slight increase in P and T wave amplitude. Moreover, qualitative analysis of ECG wave morphology and a semi-quantitative comparison is shown in Figure 3. In CP ECG (Panel A, upper panel), we observed traces with non-P wave complexes (interval in dotted line). Fully sinus conduction was observed in the CP-AV group but descending of PR interval and complex PR morphology were registered.
Figure 3. Qualitative and Semiqualitative Analysis of ECG Morphological Disturbances
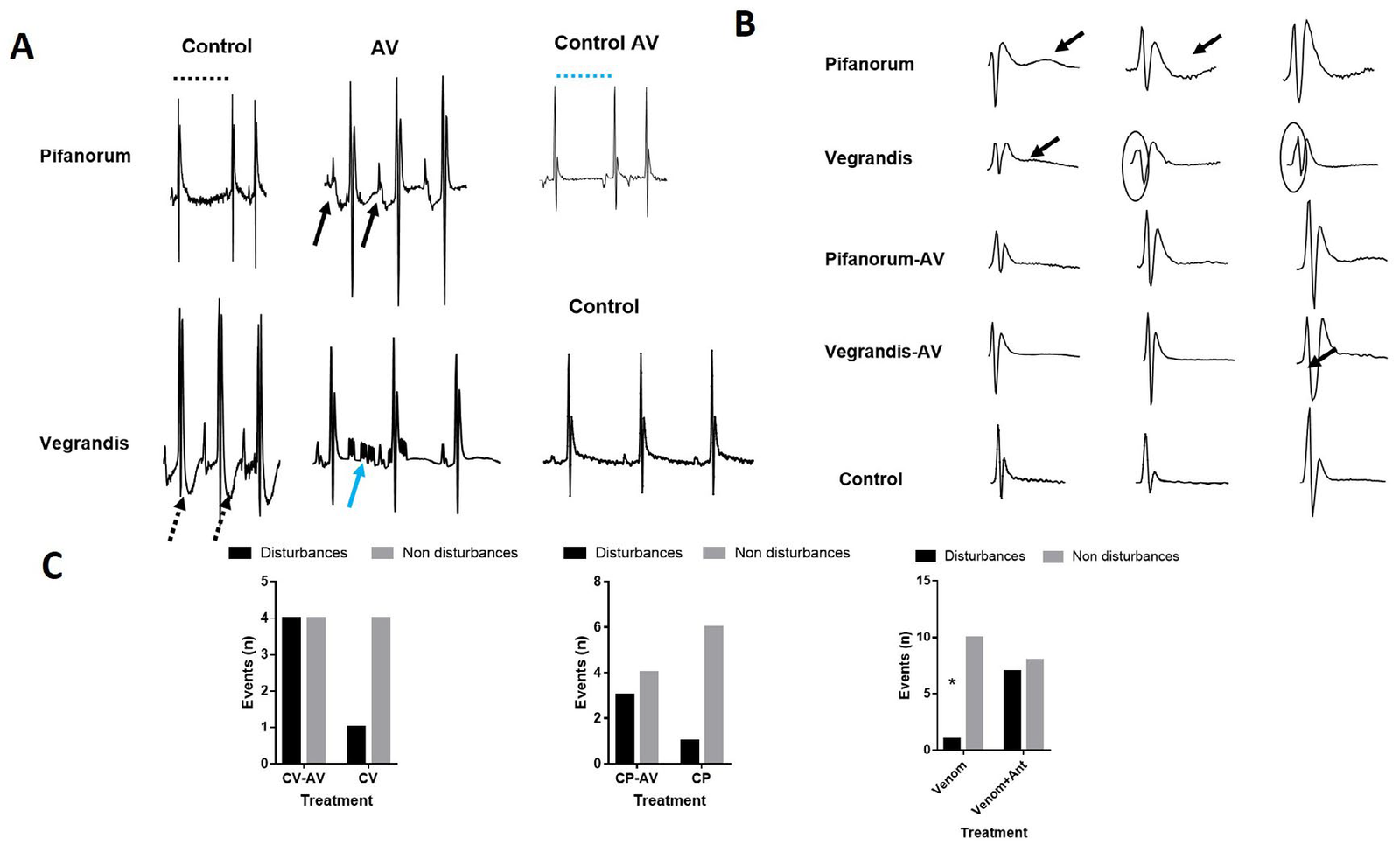
The CV panel (bottom traces), showed inversion of T wave, suggesting lesions caused by myocardial damage or myocardial ischemia. Again, in the CV-AV group, this pattern was reversed in the CV-AV group, but with increasing P morphology complexity and appearance of atrial flutter pattern (Figure 3A). Complete AV blocking was detected in traces belonging to the AV control group. Individual wave morphology showed some punctual alterations. CP recordings evidenced repolarization disorders, manifested as upright or inverted U waves (3B, upper panel). This pattern was reversed by AV incubation. The CV recordings exhibited bi-phasic T waves and QRS enlargement (3B, second-panel top-bottom sense).
Last, in panel 3C. the χ2 analysis was performed to compare the incidence of any electrocardiographic events (repolarization or conduction changes) among the different groups. Notoriously, there was a significant increase in ECG events when animals were treated with AV at any condition.
The multidimensional analysis was designed to determine the most discriminant ECG variables. Firstly, these variables contain nonredundant information and allow the identification of total differences among the groups taking the discriminant information from all variables. The model (Figure 4, Table 1) to separated CV and CP among them and between CP and CP-AV and CVCV AV. Moreover, The model described similarities in the groups treated with AV with exception of a significant difference in the CV-AV vs AV comparison (Table 1). Finally, the model identified the variables associated with heart rhythm/conduction as the most discriminant ones (Table 2).
Table 1: p values for Fisher Analysis Distances |
|
AV
|
CP |
CP-AV |
CV |
CV-AV
|
AV
|
1 |
0,0008 |
0,0854 |
0,0001 |
0,0443
|
CP
|
0,0008 |
1 |
0,0226 |
0,0102 |
0,0039
|
CP-AV
|
0,0854 |
0,0226 |
1 |
0,0038 |
0,0731
|
CV
|
0,0001 |
0,0102 |
0,0038 |
1 |
0,0011
|
CV-AV
|
0,0443 |
0,0039 |
0,0731 |
0,0011 |
1
|
p values for comparisons of fisher distances among groups were estimated, considering significantly different values lesser than 0.05. Comparisons are shown in a double-entry table and significances are highlighted in bold letters. |
Table 2: p values for Determining Discriminant Variables |
Variable
|
valor-p
|
RR Interval (s)
|
0,0012
|
Heart Rate (BPM)
|
0,0034
|
P Duration (s)
|
0,0342
|
QRS Interval (s)
|
0,0100
|
QTc (s)
|
< 0,0001
|
Tpeak Tend Interval (s)
|
< 0,0001
|
P Amplitude (mV)
|
0,2615
|
R Amplitude (mV)
|
0,9687
|
S Amplitude (mV)
|
0,0580
|
ST Height (mV)
|
0,1982
|
T Amplitude (mV)
|
0,1169
|
Redundance was estimated since variables from electrocardiographic parameters related to electrical impulse conduction and voltage. Those variables with significantly lower redundancy (bold letters) were considered discriminant by the model. p threshold was 0,05. |
Figure 4. Multivariable Linear Discriminant Analysis of Electrocardiographic Variables
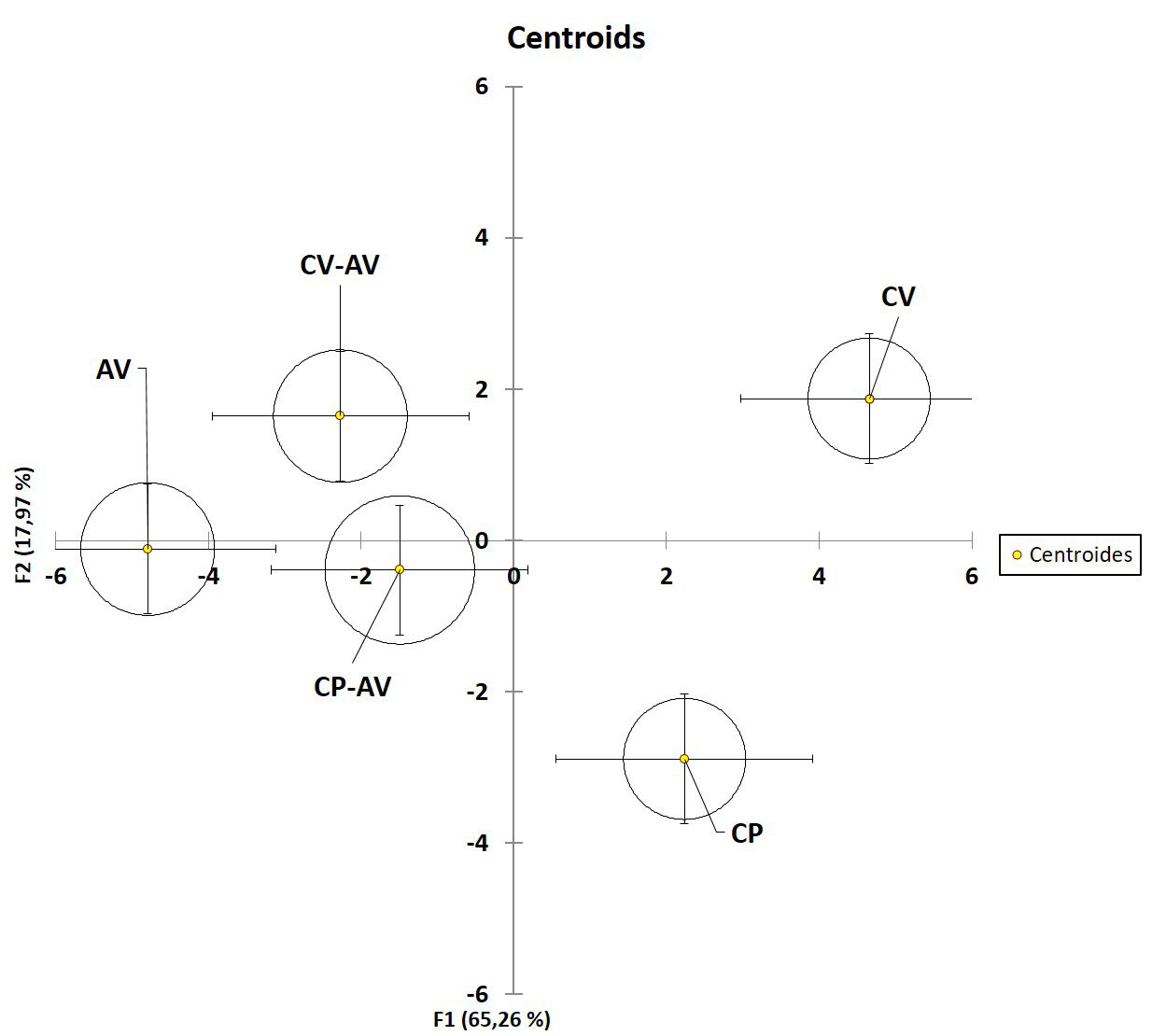
DISCUSSION
Electrophysiological disorders associated with snakebites are poorly documented and are not specifically monitored in primary care medical services. However, several cardiac disturbances have been reported for snakebites attributed to the Crotalus genus. Some authors,8 In horses, snake bitten, 40% of them were diagnosed with myocarditis, 55% with atrial premature beats, 35% with sinus pause, 40% of two or more disturbances, and to a lesser extent tachycardia ventricular and auricular. In humans, a previously elderly patient with antecedents of cardiac disease, atrial fibrillation was reported at three-hour post-crotalic envenomation,9 raising the question about the real risk of cardiac events during rattlesnake envenoming, especially for the endemic Venezuelan species included in this work with very scarce reports about the pathophysiological potential of their venom.
Slight histopathological changes were observed for C. vegrandis and C. pifanorum species (Figure 1) including tumefaction of muscle fibers in the CV inoculated group, in addition to a discrete rising of Ck-mb activity in the groups treated with antiserum. Notwithstanding, particularly for C. vegrandis, negative dromotropism, low S voltage, and T wave flattening were observed (Figure 2A). Although ST alteration segments extrapolation should be taken with caution in mice, it is possible to associate the reported changes with cardiac microcirculatory disturbances. Some human clinical reports have associated ST changes with Crotalus venoming, including infarcts in patients,14 probably linked to fibrinolytic changes associated with this kind of snakebite. This fact is interesting since C. vegrandis venom has been associated with coagulation disorders,5 similar to the pattern observed in North American species and Crotalus durissus cumanensis from some geographic regions, as potential inducers of myocardial ischemia.
Additionally, we observed in the qualitative analysis (Figure 3) T wave voltage inversion, a pattern previously associated with cardiac ischemia in murine models of cardiac disease,15 and reinforcing the association between microvascular ischemia and CV venom. The ischemia may be strongly related to mitochondrial damage reported in the literature for C. vegrandis, 6 the negative dromotropic effect (PR prolongation, Figure 2), and the cardiac fiber tumefaction observed in the histopathological analysis (Figure 1).
Following qualitative analyses, atrial ectopic activity and delayed post-depolarization were registered in both CV and CP inoculated groups (Figure 3) during the qualitative analysis. Several studies have proposed that components of Crotalus species may have potentially pro-arrhythmogenic. Crotoxin, one of the major components of the venom from the South American rattlesnake, was able to strongly potentiate L-type Ca2+ currents.16 On the other hand, crotamin, other of the principal neurotoxic components of rattlesnake venom, induced a positive chronotropic effect in skeletal muscle, probably associated with sodium activation and potassium blocker channel activity.17 Skeletal muscle studied from mice 24 h after helleramine (crotamine-like) injection showed contracted myofibrils and profound vacuolization that enlarged the subsarcolemmal space, along with loss of plasmatic and basal membrane integrity.18 Additionally, to trigger activity, the CV inoculated group also showed QRS enlargement and the effect that may be associated with myocardial ischemia discussed above.
Finally, the multiparametric analysis showed statistical differences among most groups studied. It was especially notorious between C. pifanorum and C. vegrandis groups and between the groups treated with antivenom (Figure 4, Table 1). This kind of analysis allows observing the differences of each variable, maximizing the probability of separation in a multidimensional space. In the present work, electrocardiogram variables, particularly those associated with action potential conduction (Table 2), allowed the discrimination of venom and antivenom treatment actions. These results reinforce observations that the cardiotoxicity associated with C. vegrandis venom and lesser with C. pifanorum venom may be associated with the cardiac action potential conduction. Additionally, suggests that antivenom treatment reduces the differences between C. vegrandis and pifanorum. However, the increase of arrhythmias demonstrated in the semi-quantitative analysis (Figure 3C) in groups treated with antivenom may be associated with cardiotoxic venom proteins non neutralized by commercial antivenom. Although the antivenom is made from a mixture of several Venezuelan species and in general terms was able to reverse the cardiac pathology, probably the horse immune response is not enough for generating enough neutralizing antibodies to all cardiotoxic proteins. Further research is necessary to address this issue.
CONCLUSION
In conclusion, this work was the first to demonstrate electrocardiographical disturbances following injection of C. vegrandis venom. Interestingly, these results are closely related to coagulopathy reported for this species and similar to a pro-ischemic activity reported for north-American Crotalus species. Additionally, we described triggered activity and atrial increased automaticity for both snake species venoms and demonstrated a partial neutralizing activity by commercial antivenom. This work calls attention to the necessity of performing the ECG as part of triage in patients bitten by venomous snake species. With improved ability to identify potential pro-arrhythmogenic components of the venom, we can develop better antivenom providing an improved pharmacological response to snake venom.
CONFLICTS OF INTEREST
The authors declare that they have no conflicts of interest.